Atelectasis in one lung ventilation: the good, the bad, and the ugly: a narrative review
Introduction
Rationale/background
The ways of conducting one-lung ventilation (OLV) in thoracic surgery have changed tremendously in the last four decades. Use of deleterious large tidal volumes (TVs) up to 14 mL/kg and fear of the potential hemodynamic impact of positive end-expiratory pressure (PEEP) were commonly seen in the thoracic anesthesia of the 80’s (1-3). Among important changes, optimization of lung deflation techniques, implementation of protective ventilation strategies and recognition of factors precipitating lung injury revolutionized the practice of thoracic anesthesia. Consequently, physicians should be aware of the recent advances in literature to ensure the highest standards of care for the thoracic surgery patient. They should also be informed of certain research gaps concerning techniques like PEEP titration and use of alveolar recruitment maneuvers (ARM).
Objectives
This review aims to address the recent evidence in the management of atelectasis during OLV, as it remains one of the most common complications following induction of anesthesia (4). It is of particular interest as it can become the first step in a cascade of postoperative pulmonary complications (PPCs) (5). In this case, PPCs are defined as a composite of postoperative respiratory diagnoses [atelectasis, pneumonia, and acute respiratory distress syndrome (ARDS)] that share common pathophysiological mechanisms including pulmonary collapse and airway contamination (6). Ventilation strategies that have an impact on atelectasis during the three main periods of OLV are reviewed in this article:
- Good atelectasis is the one induced in the non-ventilated (or operative) lung following lung isolation to achieve lung collapse. It is needed for thoracic surgery and is essential for the realization of video-assisted thoracic surgery (VATS) and robotic-assisted thoracic surgery (RATS). The objective of lung isolation should be to obtain a complete lung collapse of rapid onset. Here, a recently published technique is described to ensure this objective.
- During OLV, the ventilated lung (non-operative) presents a restrictive pattern of ventilation resulting from compression by both the mediastinum in lateral decubitus and the abdominal content via the inferior hemidiaphragm (7). This compression, paired with other mechanisms can precipitate atelectasis. Causes, consequences, and management of this bad atelectasis such as protective ventilation are explored in this article.
- After completion of the surgery, two-lung ventilation should be carefully re-instituted. Management of ventilation at the end of OLV is covered as it remains crucial to prevent the occurrence of any postoperative ugly atelectasis which may be responsible for PPCs.
We present this article in accordance with the Narrative Review reporting checklist (available at https://ccts.amegroups.com/article/view/10.21037/ccts-21-26/rc).
Methods
Research selection
An extended review of the relevant literature through PubMed and anesthesiology textbooks was conducted using the keywords: thoracic anesthesia, OLV, atelectasis, and PPCs (Table 1). The following study designs were included in this review: observational studies, literature reviews, randomized clinical trials, systematic reviews and meta-analyses. Scientific publications before December 31st 2022, in English or French, were considered for this review. Publications were included based on the impact they had on the management of OLV.
Table 1
Item | Specification |
---|---|
Date of search | December 31st, 2021 |
Databases and other sources searched | PubMed |
Search terms used | Thoracic anesthesia |
One lung ventilation | |
Atelectasis | |
Post-operative pulmonary complications | |
Timeframe | Before December 31st, 2021 |
Inclusion and exclusion criteria | Observational studies, literature reviews, randomized clinical trials, systematic reviews and meta-analyses were included |
Publications in English and French were considered | |
Selection process | The selection process was conducted by both authors and consensus was obtained by discussion in regard to which studies should be included |
Atelectasis: the good one
OLV represents an integral part of thoracic anesthesia especially for VATS and RATS. It is also a rare occasion in medicine where inducing atelectasis actually benefits the patient. Lung isolation with either a double-lumen endotracheal tube (DLT) or a bronchial blocker (BB) enables operative lung collapse or good atelectasis to facilitate surgical exposure of intra-thoracic structures. Ventilation of the surgical lung is interrupted by clamping the corresponding DLT lumen or by inflating the endobronchial balloon of the BB. A widespread practice amongst anesthesiologists during OLV is to open either the internal lumen of the BB or the bronchoscopy port of the DLT leading to the surgical lung to ambient air before pleural opening. The belief is that it will facilitate lung collapse by venting the lung and allowing subsequent egress of residual gas.
Over the years, many investigators published results on the efficacy of BB and DLT in the management of the OLV. A systematic review in 2014, showed that neither of the devices were superior in terms of time to achieve lung collapse (8). Most studies included in the review were of high heterogeneity and often comprised few patients. We would like to share the experience of our research program on lung collapse during OLV to address this lack in literature. Unlike other studies, our research group has obtained faster lung collapse using BB than DLT, especially when the internal channel of the BB was occluded (9,10). Based on those findings, we (11) demonstrated that a negative pressure develops in the non-ventilated surgical lung after OLV initiation and before pleural opening with both devices. This results in the entrainment of ambient air into the lung via the internal lumen of the BB or the non-ventilated lumen of the DLT if left open.
Recently, we published more results showing that the time to lung collapse could be improved if the surgical lung was excluded from communicating with ambient air before the pleura was opened (12). This study was designed with two groups and comprised only DLT. During OLV, in the control group, the DLT port leading to the surgical lung was left open before pleural opening, while it remained closed in the experimental group. Results showed that median time to lung collapse occurred faster in the closed group when compared to the open group {24 [20–37] vs. 54 [48–68] min, respectively; median difference, 30 min; 95% confidence interval (CI): 14 to 46; P=0.001}.
Therefore, rather than facilitating lung collapse in the control group, opening the bronchoscopy port of the surgical lung during closed-chest OLV allowed for ambient air entrainment. This resulted in re-nitrogenation of the lung and led to a higher residual gas volume with lower residual oxygen concentration in the lung at pleural opening. Such phenomenon lowers intra-alveolar oxygen concentration which decreases oxygen absorption by the pulmonary blood vessels and consequently reduces the speed of lung collapse. However, in the experimental group, preventing the surgical lung from communicating with ambient air before pleural opening resulted in lower residual gas volumes and a higher residual oxygen concentration inducing a faster lung collapse.
After lung resection, the remnant collapsed surgical lung should be re-expanded. It can be done with a second ventilatory circuit or with the anesthesia circuit (Figure 1) (13). Using the anesthesia circuit, the distal part of the DLT leading to the ventilated lung must be temporarily clamped to enable manual ventilation for the re-expansion of the surgical lung. This preferentially redirects the aerial flow to the collapsed surgical lung, while making sure not to fully re-expand it to avoid any forms of lung volutrauma or barotrauma (14). Manual OLV should be slow, progressive, and directed only on the surgical lung. Doing this prevents a severe diminution of venous return to the left heart since the ventilated lung is not exposed to an undesired high intra-alveolar pressure. Ideally, the inspiratory fraction of oxygen (FiO2) should be less than 0.50 thus helping recovery of the alveolar frame for the next few hours. Following manual ventilation, two lungs mechanical ventilation may be resumed with PEEP and minimally tolerated FiO2. TV should be adjusted following the amount of resected lung (e.g., a simple lobectomy equals approximately a 10–15% diminution of the TV).
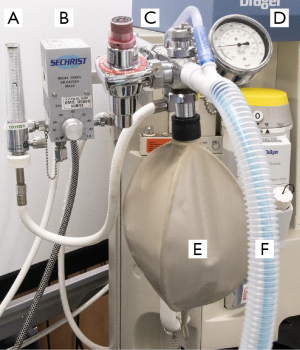
The conclusion of this research program could change the paradigm of how lung collapse is optimized during OLV. The results allow the authors to recommend routine closure of the bronchoscopy port leading to the surgical lung during closed-chest OLV. Conscientious application of this technique is warranted to obtain the expected results.
Atelectasis: the bad one
More than 90% of patients develop a certain degree of atelectasis following induction of general anesthesia (15). Since peri-operative atelectasis can trigger the installation of PPCs, it should be understood and prevented accordingly (5,16). One of the major mechanisms that promote the formation of atelectasis in the anesthetized patient is extrinsic lung compression. The sole change of position from upright to supine can decrease functional residual capacity (FRC) by about 0.7 L because of the cephalad displacement of abdominal content exerting pressure on the diaphragm (17). Induction of anesthesia leads to relaxation of the diaphragm and intercostal muscles, further reducing FRC by up to 0.8 L, leaving only half the initial volumes of an average non-obese awake patient (18). This reduction in FRC induces closure of the small airways when it falls under the closing capacity.
Resorption of alveolar gas is a second mechanism that can induce atelectasis (15). In the situation where small airways are occluded, pockets of gas distal to the occlusion will be slowly absorbed by the still perfused but non-ventilated alveoli, creating atelectasis. This may also happen in the case of intermittently closed airways where the ventilation/perfusion ratio is reduced. It must be kept in mind that the time it takes to absorb the remaining gas behind the occluded airway depends on the gas composition (19). A higher FiO2 will accelerate the resorption of gas in these alveoli since oxygen has a faster uptake than nitrogen (20).
A third mechanism involving the loss of surfactant has been proposed since mechanical ventilation with high TVs in animals can induce abnormalities in the alveolar surfactant (21). However, this mechanism may not be as contributory as compression and resorption atelectasis in OLV. Altogether, these mechanisms help to explain how atelectasis is generated during general anesthesia, creating an inflammatory reaction of the alveolus. The physiopathology of this inflammatory reaction is summarized in Figure 2.
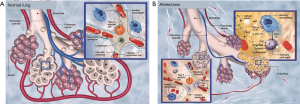
Clinical interventions to prevent atelectasis have been proposed for over 50 years in anesthesiology. In 1963, Bendixen et al. suggested that large TV and increased resistance to expiration could be means to counteract atelectasis (22). It was not until 1969 that the first clinical use of PEEP (continuous positive-pressure breathing at that time) was described in the literature (23). In 1982, the use of large TV volumes during OLV was still supported as impressive TVs of 14 mL/kg in actual body weight without any PEEP were recommended (1). Physicians feared that PEEP in OLV would have a harmful effect by diverting pulmonary flow into the non-ventilated lung thereby increasing the shunt (Figure 3) (2,3,24). This outdated belief was perpetuated in thoracic anesthesia textbooks until 2003, at which point the concept of protective ventilation started to gain recognition (25).
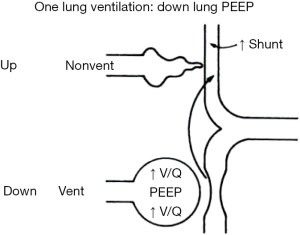
Protective ventilation
Mechanical ventilation by means of positive pressure has been found to cause lung injuries in general anesthesia patients. A combination of multiple mechanisms can explain the incidence of these injuries such as high TV causing volutrauma; high airway pressure causing barotrauma; cyclic alveolar closing and opening (atelectrauma) and a high FiO2 causing systemic inflammation (biotrauma) (26-30). Lung injuries have been found in up to 33% of patients undergoing major surgery under general anesthesia with increases in the risk of in-hospital stay, 7-day and 30-day mortality (31-34).
Thoracic surgery patients necessitating OLV are at high risk of lung injury, as all these mechanisms can affect simultaneously a single ventilated lung. In fact, this single lung is tasked with the patient’s whole respiratory load during the major part of the surgery while being exposed to a high FiO2 and the deleterious effects of mechanical ventilation. Moreover, direct injury from surgical manipulations followed by a necessary re-expansion of the non-ventilated lung results in a systemic inflammatory cascade (28,29). The lateral decubitus position required for most thoracic surgeries also induces a reduction in chest wall compliance as the ventilated lung experiences the weight of both the mediastinum and abdomen. This positioning can further contribute to atelectrauma by reducing FRC (7,26). Therefore, patients undergoing thoracic surgery represent quite a unique population with physiologic and clinical peculiarities that complexify the application of conventional lung-protective ventilation techniques (35).
The concept of protective ventilation was first described for ARDS patients in 1998 and is still part of the actual intensive care guidelines (36,37). This strategy of ventilation gave interesting results in ventilating severely pathological ARDS lungs in the intensive care units. Patients with ARDS present with stiff non-compliant lungs and impaired gas exchanges due to filling of the alveolus with inflammatory liquid (38). Even if patients requiring OLV have relatively healthy lungs, a parallel can be drawn between the two clinical situations. Both cases could be compared to a “baby lung” as the total lung surface participating in gas exchanges is greatly reduced and may be submitted to an excessive mechanical stress from ventilation (39). In fact, the protective ventilation strategy of ARDS was quicky adopted in anesthesia to minimize the pulmonary effect of ventilation on healthy lungs in the operating room. Protective ventilation was first tested and adopted during abdominal surgery at the beginning of 2000 (40). It took a few more years to accept this practice in thoracic surgery. Changes occurred mainly after the publication by Michelet et al. presenting favorable results after esophagectomy (41). Low TV of 5 mL/kg with a PEEP of 5 cmH2O was shown to improve lung function and decreased both the proinflammatory systemic response and time to extubation when compared to a TV of 9 mL/kg without PEEP (41). Those results triggered a change of practice towards the use of protective ventilation in the operating room, notably in OLV.
Protective ventilation in OLV includes two main modalities: low TV, the use of PEEP and according to certain recommendations, ARM (42). A majority of published studies recommend TV during OLV to be from 4 to 6 mL/kg of ideal body weight, based on the ARDS net formula (43) or the body mass index (BMI) (44). The objective in using low TV is to prevent PPCs by minimizing volutrauma, barotrauma, and biotrauma (45). Application of PEEP during OLV helps to restore functional FRC, therefore avoiding closure of the small airways. This also stabilizes the alveolus by avoiding cyclic opening and closure, which decreases shear stress and prevents alveolar collapse leading to atelectrauma (15).
PEEP needs to be titrated, as too little is ineffective, and too much increases the shunt through the non-ventilated lung. Ideally, the level of PEEP should be selected by challenging either the dynamic compliance (32) or the driving pressure (plateau pressure minus PEEP) of the lung (46). For instance, the dynamic compliance of the lung is defined as the change in TV divided by the change in airway pressure. Dynamic compliance can be measured during mechanical ventilation and mainly depends on the compliance of the chest wall, lung tissue and airway resistance. A decremental or incremental titration of PEEP helps determining the highest dynamic compliance after lung recruitment, improving oxygenation and lung mechanics (47). Also, titration of PEEP to produce the lowest driving pressure showed reduction in postoperative pneumonia or ARDS in a recent study on OLV (P=0.028, odds ratio 0.42; 95% CI: 0.19 to 0.92) (46). Beyond these benefits, the effect of an individualized PEEP on patient outcomes remains mostly unknown and warrants further clinical studies. Additionally, ARM aim to reverse intra-operative atelectasis using brief and controlled increases in airway pressure with the expansion of the lung (48,49).
Major randomized controlled trials comparing conventional and protective ventilation during OLV have been published in the last two decades. Two recent and well-designed systematic reviews and meta-analyses evaluated ventilatory strategies during OLV (26,50). The conclusions of this extensive work are summarized in Figures 4,5. The first publication (50) evaluated low vs. conventional TV during OLV (5.6±0.8 vs. 8.1±3.1 mL/kg). Despite concerns of intraoperative hypoventilation, the evidence suggested no worsening of oxygenation or pulmonary compliance low TV. Patients in the low TV group showed a significant reduction in PPC [pooled odds ratio, 0.40 (0.29–0.57); P<0.0001]. This evidence supports lung protective ventilation strategies and the necessity of individualizing TV based on ideal weight for each patient undergoing OLV.
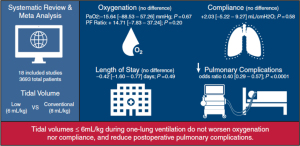
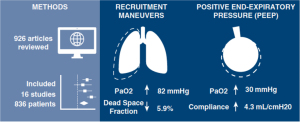
The other systematic review and meta-analysis from the same group of authors (26) assessed the existing evidence regarding the use of PEEP and ARM during lung-protective ventilation in OLV. PEEP and ARM were associated with significant improvements in partial pressure of oxygen (PaO2) during OLV, suggesting that recruitment maneuvers improve PaO2. However, their optimal use needs to be further investigated as only physiological benefits have been reported with PEEP titration and ARM in OLV (26). A possible explanation for the lack of clinical benefits may be the context in which PEEP is studied. When titrated as a sole parameter of ventilation, benefits may only be physiological, but when titrated concomitantly with airway plateau pressures to obtain the lowest driving pressure, it could benefit clinical outcomes. As mentioned previously, driving pressure which takes PEEP into account, may become a valid predictor of PPCs in the upcoming literature (32).
Recently, interrogations about potential deleterious effects of ARM during OLV for thoracic surgery were raised. Data suggests a potentially harmful effect of recruitment: immediate physiologic parameters may be improved, but this transitory effect may come at the expense of delayed lung inflammation/injury (49). Two major multicentric European studies, Prothor, and iProve-OLV, which were launched a few years ago and are still ongoing, are examining the use of protective ventilation during OLV (51,52). Prothor aims to study the impact of a high PEEP with ARM vs. low PEEP without ARM, while iProve-OLV aims to study ARM with individualized PEEP vs. conventional protective ventilation. These studies should bring interesting data regarding the clinical value of ARM as a strategy of ventilation during OLV. In the meantime, the relevance of ARM should be adapted to each clinical situation. Finally, we propose an overview of the desaturation management during OLV that includes both PEEP and ARM as strategies to optimize oxygenation (53) (Table 2).
Table 2
General maneuvers |
FiO2 of 100% |
Assessment of the pulmonary isolation |
Spirometry |
Expiratory volumes |
Seal of the bronchial cuff |
Video-bronchoscopy |
Isolation device positioning |
Suctioning of secretions if necessary |
Cardiac output |
Vasopressors, inotropes |
Lower anesthetic gas concentration |
Maneuvers on the dependent ventilated lung |
PEEP titration |
Minute ventilation adjustment |
Recruitment maneuvers |
Maneuvers on the non-dependent surgical lung |
Oxygenation |
O2 insufflation |
CPAP 3–5–10 cmH2O |
Intermittent ventilation |
Pulmonary artery clamping (only in extreme cases) |
Reference adapted from: Cournoyer C, Bussieres J. Chapter 23: Respiratory System and Anesthesia. In: Beaulieu P. editor. Handbook of Anesthesia and Intensive Care. 6th edition. Montreal: Montréal University Press, 2020;456-75. FiO2, fraction of inspired oxygen; PEEP, positive end-expiratory pressure; CPAP, continuous positive airway pressure.
Atelectasis: “the ugly one”
PPCs are always dreaded in the postoperative course of lung surgery. They need to be prevented and treated aggressively as they are reported in as much as 7% to 49% of cases with an associated mortality ranging between 2% to 12% (54). Despite continuous improvements in the operating room and post-operative care, they continue to pose a serious threat to successful outcomes. The persistence of atelectasis from the perioperative to the postoperative setting can represent the starting point for a deleterious cascade of PPCs (16). In this third part, we will detail the occurrence of this ugly atelectasis when looking at surgical approaches to the thoracic cavity and oxygen concentration used during maintenance and emergence of anesthesia. Of note, ugly atelectasis may also originate from airway obstruction (secretions) and altered respiratory mechanics often aggravated by a sub-optimal analgesia. Analgesic modalities will be discussed in a specific article of this series “Recent Advances in Perioperative Care in Thoracic Surgery and Anesthesia” (Clairoux A, Issa R, Bélanger MÈ, et al. Perioperative pain management for thoracic surgery: a narrative review of the literature. Curr Chall Thorac Surg 2023;5:36).
Surgical approaches
The development of new technologies, like VATS, to perform lung resection helped to reduce the incidence of PPCs. When compared to thoracotomy, VATS was associated with earlier mobilization, better preservation of pulmonary function, and shorter length-of-stay (55-57). A large propensity-matched study from the European Society of Thoracic Surgeons database consisting of 28,771 patients showed a significant reduction in total postoperative complications, major cardiopulmonary complications, atelectasis requiring bronchoscopy, and wound infection in favor of VATS (58). The clinical benefits of a minimally invasive approach are particularly evident in high-risk patients with poor predicted postoperative lung function (59). Another advantage of VATS against the open thoracotomy is also the minimal intensity and duration of postoperative pain (56).
One of the most recent advances in lung surgery is the non-intubated (awake) VATS (NIVATS). A recent propensity score-matched analysis comparing NIVATS and intubated VATS showed a reduction in operative and anesthesia times with a faster and more stable recovery in the postoperative care unit. However, in an immediate postoperative setting, chest radiography showed higher rates of pulmonary atelectasis in the NIVATS group than in the standard VATS group (16.0% vs. 2.5%, P<0.001). A follow-up of the radiological evolution through time would have been interesting as the NIVATS group may have had a faster respiratory recovery (60). Further evaluation with multi-centric, prospective, and large-cohort clinical trials is required to evaluate the different effects of this new surgical and anesthetic technique.
Oxygen concentration
Supplemental oxygen is mandatory in the context of general anesthesia. A meticulous preoxygenation is done before induction to achieve an oxygen reserve in case of an unexpected difficult airway and to provide time for the complexity of DLT insertion and positioning. Following induction, an FiO2 of 1.0 is used before the beginning of OLV to promote absorption atelectasis and increase the quality of lung deflation, facilitating surgical acts. The increase in FiO2 also serves to counteract the consequences of the shunt caused by both atelectasis in the dependent lung and blood flow perfusing the non-dependent lung. Despite those advantages, the non-judicious use of supplemental oxygen may prove to be detrimental for the thoracic surgery patient, and the anesthesiologist should try to decrease progressively the FiO2. The use of a higher FiO2 during OLV may indeed be associated with an increased incidence of PPCs by means of direct pulmonary toxicity (61). The now recognized toxicity of oxygen could therefore play a role in the postoperative course of a patients who undergo OLV (62).
Moreover, the use of a high FiO2 during induction, maintenance of OLV, and emergence is a major cause of anesthesia-induced atelectasis, which is secondary to alveolar gas resorption (63,64). An interesting study, although not on OLV, showed that lung recruitment with a FiO2 of 1.0 during two lung ventilation resulted in the rapid reappearance of atelectasis when compared to a FiO2 of 0.4 because of absorption atelectasis (65). This demonstration could theoretically be applied as well in OLV. Using the lowest possible intra-operative FiO2 raises the inspired concentration of nitrogen and may help to minimize the recurrence of atelectasis. Thus, ARMs must be followed by ventilation with moderate FiO2 (19). In our experience, an FiO2 of 0.40–0.50 during OLV should suffice if the ventilated lung is not atelectatic and if the patient medical condition allows it. Supplemental oxygenation remains an essential tool in the management of the anesthetized thoracic patient, but it must be kept in mind that even this daily essential can become harmful in excess.
Limitations
Although multiple authors have researched on the optimal management in OLV, there remains a need for solid randomized controlled trials examining key features like protective ventilation and recruitment maneuvers in OLV. Our review is therefore limited by our access to such studies and the need to gather information from smaller trials. Those large scale, multi-centric studies evaluating ventilatory strategies like Prothor and iProve-OLV will follow in the next years (51,52). In the future, studies should try to evaluate separately the modalities of protective ventilation to assess the impact of each of them. This could lead to an individualization of ventilatory parameters in OLV benefiting the thoracic surgery patient.
Conclusions
In summary, OLV represents a ventilatory challenge for both the thoracic surgery patient and anesthesiologist. In such conditions, small details may matter more than we think. Altogether FiO2, TV, PEEP, and possibly the use of ARMs should be meticulously optimized for each patient. As of today, it remains hard to see if these individual components benefit the patient, however, studies tend to go in the same direction for each one of them. All things considered, the management of OLV during thoracic surgery has changed tremendously in the last 20 years. We hope this review gave you insights on how to optimize good atelectasis for an easier surgical resection, to preserve the ventilated lung from bad atelectasis leading to desaturation, and to minimize the installation of ugly atelectasis hereby preventing postoperative PPCs. The main objective of the anesthetic management should be to keep the lung open and to deliver the patient to the postoperative ward with the least amount of atelectasis (63).
Acknowledgments
Portions of this article are based on a presentation at the French Society of Anesthesia and Intensive Care (SFAR) annual meeting 2019, Paris, France.
Funding: None.
Footnote
Provenance and Peer Review: This article was commissioned by the editorial office, Current Challenges in Thoracic Surgery for the series “Recent Advances in Perioperative Care in Thoracic Surgery and Anesthesia”. The article has undergone external peer review.
Reporting Checklist: The authors have completed the Narrative Review reporting checklist. Available at: https://ccts.amegroups.com/article/view/10.21037/ccts-21-26/rc
Peer Review File: Available at: https://ccts.amegroups.com/article/view/10.21037/ccts-21-26/prf
Conflicts of Interest: Both authors have completed the ICMJE uniform disclosure form (available at: https://ccts.amegroups.com/article/view/10.21037/ccts-21-26/coif). The series “Recent Advances in Perioperative Care in Thoracic Surgery and Anesthesia” was commissioned by the editorial office without any funding or sponsorship. JSB served as the unpaid Guest Editor of the series. The authors have no other conflicts of interest to declare.
Ethical Statement: The authors are accountable for all aspects for the work in ensuring that questions related to the accuracy or integrity of any part of the work are appropriately investigated and resolved.
Open Access Statement: This is an Open Access article distributed in accordance with the Creative Commons Attribution-NonCommercial-NoDerivs 4.0 International License (CC BY-NC-ND 4.0), which permits the non-commercial replication and distribution of the article with the strict proviso that no changes or edits are made and the original work is properly cited (including links to both the formal publication through the relevant DOI and the license). See: https://creativecommons.org/licenses/by-nc-nd/4.0/.
References
- Katz JA, Laverne RG, Fairley HB, et al. Pulmonary oxygen exchange during endobronchial anesthesia: effect of tidal volume and PEEP. Anesthesiology 1982;56:164-71. [Crossref] [PubMed]
- Capan LM, Turndorf H, Patel C, et al. Optimization of arterial oxygenation during one-lung anesthesia. Anesth Analg 1980;59:847-51. [Crossref] [PubMed]
- Tarhan S, Lundborg RO. Effects of increased expiratory pressure on blood gas tensions and pulmonary shunting during thoracotomy with use of the Carlens catheter. Can Anaesth Soc J 1970;17:4-11. [Crossref] [PubMed]
- Hedenstierna G, Rothen HU. Atelectasis formation during anesthesia: causes and measures to prevent it. J Clin Monit Comput 2000;16:329-35. [Crossref] [PubMed]
- Duggan M, Kavanagh BP. Atelectasis in the perioperative patient. Curr Opin Anaesthesiol 2007;20:37-42. [Crossref] [PubMed]
- Abbott TEF, Fowler AJ, Pelosi P, et al. A systematic review and consensus definitions for standardised end-points in perioperative medicine: pulmonary complications. Br J Anaesth 2018;120:1066-79. [Crossref] [PubMed]
- Ng A, Swanevelder J. Hypoxaemia during one-lung anaesthesia. Continuing Education in Anaesthesia Critical Care & Pain 2010;10:117-22. [Crossref]
- Clayton-Smith A, Bennett K, Alston RP, et al. A Comparison of the Efficacy and Adverse Effects of Double-Lumen Endobronchial Tubes and Bronchial Blockers in Thoracic Surgery: A Systematic Review and Meta-analysis of Randomized Controlled Trials. J Cardiothorac Vasc Anesth 2015;29:955-66. [Crossref] [PubMed]
- Bussières JS, Somma J, Del Castillo JL, et al. Bronchial blocker versus left double-lumen endotracheal tube in video-assisted thoracoscopic surgery: a randomized-controlled trial examining time and quality of lung deflation. Can J Anaesth 2016;63:818-27. [Crossref] [PubMed]
- Cohen E. Strategies for lung isolation: to block or not to block? Can J Anaesth 2016;63:797-801. [Crossref] [PubMed]
- Moreault O, Couture EJ, Provencher S, et al. Double-lumen endotracheal tubes and bronchial blockers exhibit similar lung collapse physiology during lung isolation. Can J Anaesth 2021;68:791-800. [Crossref] [PubMed]
- Somma J, Couture ÉJ, Pelletier S, et al. Non-ventilated lung deflation during one-lung ventilation with a double-lumen endotracheal tube: a randomized-controlled trial of occluding the non-ventilated endobronchial lumen before pleural opening. Can J Anaesth 2021;68:801-11. [Crossref] [PubMed]
- Bussières J, Cournoyer C, Couture EJ. Use of a second ventilatory circuit when using a double-lumen endotracheal tube. Can J Anaesth 2020;67:1114-5. [Crossref] [PubMed]
- Lohser J, Slinger P. Lung Injury After One-Lung Ventilation: A Review of the Pathophysiologic Mechanisms Affecting the Ventilated and the Collapsed Lung. Anesth Analg 2015;121:302-18. [Crossref] [PubMed]
- Duggan M, Kavanagh BP. Pulmonary atelectasis: a pathogenic perioperative entity. Anesthesiology 2005;102:838-54. [Crossref] [PubMed]
- Tusman G, Böhm SH, Warner DO, et al. Atelectasis and perioperative pulmonary complications in high-risk patients. Curr Opin Anaesthesiol 2012;25:1-10. [Crossref] [PubMed]
- Ibañez J, Raurich JM. Normal values of functional residual capacity in the sitting and supine positions. Intensive Care Med 1982;8:173-7. [Crossref] [PubMed]
- Juno J, Marsh HM, Knopp TJ, et al. Closing capacity in awake and anesthetized-paralyzed man. J Appl Physiol Respir Environ Exerc Physiol 1978;44:238-44. [Crossref] [PubMed]
- Hedenstierna G, Edmark L. Mechanisms of atelectasis in the perioperative period. Best Pract Res Clin Anaesthesiol 2010;24:157-69. [Crossref] [PubMed]
- Joyce CJ, Baker AB, Kennedy RR. Gas uptake from an unventilated area of lung: computer model of absorption atelectasis. J Appl Physiol (1985) 1993;74:1107-16. [Crossref] [PubMed]
- Dreyfuss D, Saumon G. Ventilator-induced lung injury: lessons from experimental studies. Am J Respir Crit Care Med 1998;157:294-323. [Crossref] [PubMed]
- Bendixen HH, Hedley-Whyte J, Laver MB. Impaired oxygenation in surgical patients during general anesthesia with controlled ventilation. a concept of atelectasis. N Engl J Med 1963;269:991-6. [Crossref] [PubMed]
- Ashbaugh DG, Petty TL, Bigelow DB, et al. Continuous positive-pressure breathing (CPPB) in adult respiratory distress syndrome. J Thorac Cardiovasc Surg 1969;57:31-41. [Crossref] [PubMed]
- Triantafillou AN, Benumof JL, Lecamwasam HS. Chapter 4: Physiology of the Lateral Decubitus Position, the Open Chest, and One Lung Ventilation. In: Kaplan JS, Slinger PD. editors. Thoracic Anesthesia. 3rd edition. Philadelphia: Churchill Livingstone, 2003:87 (Fig 4-17).
- Kaplan JS, Slinger PD. Physiology of the Lateral Decubitus, the Open Chest, and One Lung Ventilation. Thoracic Anesthesia. 3rd edition. Philadelphia: Churchill Livingstone; 2003.
- Peel JK, Funk DJ, Slinger P, et al. Positive end-expiratory pressure and recruitment maneuvers during one-lung ventilation: A systematic review and meta-analysis. J Thorac Cardiovasc Surg 2020;160:1112-1122.e3. [Crossref] [PubMed]
- Curley GF, Laffey JG, Zhang H, et al. Biotrauma and Ventilator-Induced Lung Injury: Clinical Implications. Chest 2016;150:1109-17. [Crossref] [PubMed]
- Uhlig S. Ventilation-induced lung injury and mechanotransduction: stretching it too far? Am J Physiol Lung Cell Mol Physiol 2002;282:L892-6. [Crossref] [PubMed]
- Della Rocca G, Coccia C. Acute lung injury in thoracic surgery. Curr Opin Anaesthesiol 2013;26:40-6. [Crossref] [PubMed]
- Williams EA, Quinlan GJ, Goldstraw P, et al. Postoperative lung injury and oxidative damage in patients undergoing pulmonary resection. Eur Respir J 1998;11:1028-34. [Crossref] [PubMed]
- Fernandez-Bustamante A, Frendl G, Sprung J, et al. Postoperative Pulmonary Complications, Early Mortality, and Hospital Stay Following Noncardiothoracic Surgery: A Multicenter Study by the Perioperative Research Network Investigators. JAMA Surg 2017;152:157-66. [Crossref] [PubMed]
- Khuri SF, Henderson WG, DePalma RG, et al. Determinants of long-term survival after major surgery and the adverse effect of postoperative complications. Ann Surg 2005;242:326-41; discussion 341-3. [Crossref] [PubMed]
- Serpa Neto A, Cardoso SO, Manetta JA, et al. Association between use of lung-protective ventilation with lower tidal volumes and clinical outcomes among patients without acute respiratory distress syndrome: a meta-analysis. JAMA 2012;308:1651-9. [Crossref] [PubMed]
- Lellouche F, Dionne S, Simard S, et al. High tidal volumes in mechanically ventilated patients increase organ dysfunction after cardiac surgery. Anesthesiology 2012;116:1072-82. [Crossref] [PubMed]
- Kutlu CA, Williams EA, Evans TW, et al. Acute lung injury and acute respiratory distress syndrome after pulmonary resection. Ann Thorac Surg 2000;69:376-80. [Crossref] [PubMed]
- Amato MB, Barbas CS, Medeiros DM, et al. Effect of a protective-ventilation strategy on mortality in the acute respiratory distress syndrome. N Engl J Med 1998;338:347-54. [Crossref] [PubMed]
- Papazian L, Aubron C, Brochard L, et al. Formal guidelines: management of acute respiratory distress syndrome. Ann Intensive Care 2019;9:69. [Crossref] [PubMed]
- Meyer NJ, Gattinoni L, Calfee CS. Acute respiratory distress syndrome. Lancet 2021;398:622-37. [Crossref] [PubMed]
- Sentürk M. New concepts of the management of one-lung ventilation. Curr Opin Anaesthesiol 2006;19:1-4. [Crossref] [PubMed]
- Wrigge H, Uhlig U, Zinserling J, et al. The effects of different ventilatory settings on pulmonary and systemic inflammatory responses during major surgery. Anesth Analg 2004;98:775-81. table of contents. [Crossref] [PubMed]
- Michelet P, D'Journo XB, Roch A, et al. Protective ventilation influences systemic inflammation after esophagectomy: a randomized controlled study. Anesthesiology 2006;105:911-9. [Crossref] [PubMed]
- Piccioni F, Droghetti A, Bertani A, et al. Recommendations from the Italian intersociety consensus on Perioperative Anesthesa Care in Thoracic surgery (PACTS) part 2: intraoperative and postoperative care. Perioper Med (Lond) 2020;9:31. [Crossref] [PubMed]
- Acute Respiratory Distress Syndrome Network. Ventilation with lower tidal volumes as compared with traditional tidal volumes for acute lung injury and the acute respiratory distress syndrome. N Engl J Med 2000;342:1301-8. [Crossref] [PubMed]
- Moreault O, Lacasse Y, Bussières JS. Calculating Ideal Body Weight: Keep It Simple. Anesthesiology 2017;127:203-4. [Crossref] [PubMed]
- Yang M, Ahn HJ, Kim K, et al. Does a protective ventilation strategy reduce the risk of pulmonary complications after lung cancer surgery?: a randomized controlled trial. Chest 2011;139:530-7. [Crossref] [PubMed]
- Park M, Ahn HJ, Kim JA, et al. Driving Pressure during Thoracic Surgery: A Randomized Clinical Trial. Anesthesiology 2019;130:385-93. [Crossref] [PubMed]
- Rauseo M, Mirabella L, Grasso S, et al. Peep titration based on the open lung approach during one lung ventilation in thoracic surgery: a physiological study. BMC Anesthesiol 2018;18:156. [Crossref] [PubMed]
- Lohser J, Ishikawa S. Clinical Management of One-Lung Ventilation. In: Springer, editor. Principles and Practice of Anesthesia for Thoracic Surgery. 1st edition. New-York: Springer, 2011:83-101.
- Kidane B, Palma DC, Badner NH, et al. The Potential Dangers of Recruitment Maneuvers During One Lung Ventilation Surgery. J Surg Res 2019;234:178-83. [Crossref] [PubMed]
- Peel JK, Funk DJ, Slinger P, et al. Tidal volume during 1-lung ventilation: A systematic review and meta-analysis. J Thorac Cardiovasc Surg 2022;163:1573-1585.e1. [Crossref] [PubMed]
- Kiss T, Wittenstein J, Becker C, et al. Protective ventilation with high versus low positive end-expiratory pressure during one-lung ventilation for thoracic surgery (PROTHOR): study protocol for a randomized controlled trial. Trials 2019;20:213. [Crossref] [PubMed]
- Carramiñana A, Ferrando C, Unzueta MC, et al. Rationale and Study Design for an Individualized Perioperative Open Lung Ventilatory Strategy in Patients on One-Lung Ventilation (iPROVE-OLV). J Cardiothorac Vasc Anesth 2019;33:2492-502. [Crossref] [PubMed]
- Cournoyer C, Bussieres J. Chapter 23: Respiratory System and Anesthesia. In: Beaulieu P. editor. Handbook of Anesthesia and Intensive Care. 6th editor. Montreal: Montréal University Press, 2020;456-75.
- Stéphan F, Boucheseiche S, Hollande J, et al. Pulmonary complications following lung resection: a comprehensive analysis of incidence and possible risk factors. Chest 2000;118:1263-70. [Crossref] [PubMed]
- Rogers LJ, Bleetman D, Messenger DE, et al. The impact of enhanced recovery after surgery (ERAS) protocol compliance on morbidity from resection for primary lung cancer. J Thorac Cardiovasc Surg 2018;155:1843-52. [Crossref] [PubMed]
- Cao C, Manganas C, Ang SC, et al. Video-assisted thoracic surgery versus open thoracotomy for non-small cell lung cancer: a meta-analysis of propensity score-matched patients. Interact Cardiovasc Thorac Surg 2013;16:244-9. [Crossref] [PubMed]
- Batchelor TJP, Rasburn NJ, Abdelnour-Berchtold E, et al. Guidelines for enhanced recovery after lung surgery: recommendations of the Enhanced Recovery After Surgery (ERAS®) Society and the European Society of Thoracic Surgeons (ESTS). Eur J Cardiothorac Surg 2019;55:91-115. [Crossref] [PubMed]
- Falcoz PE, Puyraveau M, Thomas PA, et al. Video-assisted thoracoscopic surgery versus open lobectomy for primary non-small-cell lung cancer: a propensity-matched analysis of outcome from the European Society of Thoracic Surgeon database. Eur J Cardiothorac Surg 2016;49:602-9. [Crossref] [PubMed]
- Burt BM, Kosinski AS, Shrager JB, et al. Thoracoscopic lobectomy is associated with acceptable morbidity and mortality in patients with predicted postoperative forced expiratory volume in 1 second or diffusing capacity for carbon monoxide less than 40% of normal. J Thorac Cardiovasc Surg 2014;148:19-28, dicussion 28-29.e1.
- Lan L, Cen Y, Zhang C, et al. A Propensity Score-Matched Analysis for Non-Intubated Thoracic Surgery. Med Sci Monit 2018;24:8081-7. [Crossref] [PubMed]
- Okahara S, Shimizu K, Suzuki S, et al. Associations between intraoperative ventilator settings during one-lung ventilation and postoperative pulmonary complications: a prospective observational study. BMC Anesthesiol 2018;18:13. [Crossref] [PubMed]
- Lumb AB, Walton LJ. Perioperative oxygen toxicity. Anesthesiol Clin 2012;30:591-605. [Crossref] [PubMed]
- Hedenstierna G. Oxygen and anesthesia: what lung do we deliver to the post-operative ward? Acta Anaesthesiol Scand 2012;56:675-85. [Crossref] [PubMed]
- Restrepo RD, Braverman J. Current challenges in the recognition, prevention and treatment of perioperative pulmonary atelectasis. Expert Rev Respir Med 2015;9:97-107. [Crossref] [PubMed]
- Benoît Z, Wicky S, Fischer JF, et al. The effect of increased FIO(2) before tracheal extubation on postoperative atelectasis. Anesth Analg 2002;95:1777-81. table of contents. [Crossref] [PubMed]
Cite this article as: Bussières JS, Marques E. Atelectasis in one lung ventilation: the good, the bad, and the ugly: a narrative review. Curr Chall Thorac Surg 2023;5:38.