Ablation for treating small pulmonary nodules: radiologic and surgical approach and perspectives
Introduction
The leading cause of cancer-related mortality in the United States is lung cancer. Lung cancer has a projected incidence in 2022 of 236,740 cases and 130,180 deaths and non-small cell lung cancer (NSCLC) accounts for approximately 85% of cases (1). Surgical resection is the standard of care for early-stage NSCLC. However, a significant proportion of early-stage lung cancer patients may not be surgical candidates due to medical comorbidities (2,3). The American Association for Thoracic Surgery (AATS) recently published an expert panel consensus document on the assessment of high-risk for lobectomy in patients with stage I NSCLC. Patients with early-stage lung cancer who are not surgical candidates are traditionally offered chemotherapy and radiation, treatments that have been associated with relatively modest improvements in survival.
In patients, who are medically inoperable or considered high-risk for complications after surgery, percutaneous image-guided ablation is a safe, cost-effective, minimally invasive treatment alternative. Image-guided tumor ablation procedures use extreme hot or cold temperatures to destroy tumors. Image-guided tumor ablation may be used alone for tumors that are not amenable for surgical treatment or in patients who are not surgical candidates such as elderly individuals with frailty. It may also be used as an adjunct to surgery, chemotherapy, or radiation, as a curative therapy, and as a palliative therapy. Ablative techniques, including radiofrequency ablation (RFA), may be applicable in high-risk patients with lung cancer (4).
RFA is the most studied ablative treatment option for lung cancer. Treating lung cancer with RFA was one of the first uses of ablative therapies in the lung, and the procedure has undergone significant advances since it was first described in 2000 (5). Advances have included technical innovations, improved approaches, careful patient selection, and enhanced understanding of features seen in preoperative and postoperative imaging. In selected patients, image-guided tumor ablation is a well-recognized treatment option for patients who are poor surgical candidates or who refuse external beam radiotherapy and the indications for ablative therapies are expanding as the effectiveness of ablative therapies improves.
In this paper, the indications for lung ablative procedures and the techniques of ablation, with a focus on RFA and its role in the management of primary lung carcinoma, pulmonary metastases, and recurrent lung neoplasm are reviewed.
Indications for ablation of thoracic neoplasms
Ablation is indicated for the treatment of selected patients with primary lung cancer, lung metastases, or recurrent lung neoplasm after surgery or other therapy. Oligometastatic disease is one of the most common indications for lung ablation. Importantly, ablation provides an option that is repeatable, and is associated with minimal loss of pulmonary function. Since 2009, extensive publications have documented the safety and efficacy of image-guided tumor ablation (6). Thermal ablation is also cost effective. Alexander and colleagues conducted a retrospective analysis of 84 patients older than 65 years of age with stage I NSCLC treated with RFA (n=56) or sublobar resection (n=28) (7). Patients treated with RFA were older, and mortality was significantly lower in patients treated with sublobar resection. However, the median cost per month lived for patients treated with RFA was $620.74 and was significantly lower than the cost per month lived in patients treated with sublobar resection ($1,195.92) (P=0.0002). While the authors concluded that patients treated with surgery had a significantly improved survival, they also noted that RFA provides an alternative treatment option at a significantly lower cost for patients who are not surgical candidates. In another study, Kwan and colleagues used propensity-score matching to compare the medical costs of treatment of early-stage NSCLC between thermal ablation and sublobar resection using the Surveillance, Epidemiology, and End Results (SEER) database and Medicare-linked data (8). They reported that patients treated with ablation had significantly lower treatment-related costs as compared with those treated with sublobar resection (P<0.001). These studies suggest that in selected patients, RFA is cost-effective as compared with sublobar resection.
Ablation techniques
Current image-guided tumor ablation modalities for treating lung tumors include RFA, cryoablation (CA) and microwave ablation (MWA). RFA and MWA induce tissue injury by increasing tissue temperature. In contrast, CA cools tissue to cytotoxic temperatures. The choice of the modality of ablation can depend on many factors including tumor size and location. Each technique has specific advantages and disadvantages.
Principles of RFA
RFA was the first image-guided tumor ablation technique applied to lung tumors and primary lung cancer (6,9). A radiofrequency energy generator supplies an alternating current that is delivered through a needle electrode (4). The electrode is most often introduced into the tumor percutaneously under computed tomography (CT) guidance. The alternating electrical current, in the frequency of radio waves, generates frictional heat as high as the boiling point of water from the ionic agitation. This high heat in the region of the electrode leads to coagulative tissue necrosis (4). Advantages of RFA are that it is supported by years of experience, has well-established safety and efficacy, and is readily available at most centers. Because the user can gauge the temperature, it also allows for precise treatment with less likelihood of over-treatment.
Minor limitations of RFA include a slightly longer set-up time, high tissue impedance that may limit the size of the ablated area, and rarely potential interference with the cardiac conduction system and pacemakers within the current pathway. In addition, the cooling effect of flowing blood in large blood vessels and of air in well-perfused and aerated lung tissue can impede thermal ablation. These heat-sink effects may lower the extent of ablation achievable and prevent destruction of adjacent tumor tissue. RFA is contraindicated in the central third of the lung close to the hilum near the central vessels and airways.
MWA
MWA uses electromagnetic microwave radiation to increase tissue temperature and induce cell injury and death. MWA has some potential advantages over RFA. MWA can generate higher temperatures because it utilizes a much higher frequency, and potentially larger ablation zones. MWA is preferred for larger tumors, as multiple antennae allow for ablation zones of up to 6 cm (10). The procedure time is shorter, and it is also safer in patients with cardiac devices.
The limitations of MWA are mainly related to difficulty in accurately gauging temperature, which may cause over-treatment and lack of clinical experience with current systems (11). To obtain predictable and safe results, users should become familiar with the technical parameters of the MWA procedure including power, time allocation, antennae type, and frequency ranges.
CA
CA induces cell death through subzero temperatures below 20–40 degrees Celsius. Pressurized gas, most commonly argon, is introduced through a closed sealed cryoprobe. A zone of freezing or “ice ball” with a gradient of “isotherms” or constant temperature zones can be achieved. The temperature may be as low as low as −170 ℃ centrally and as high as 0 ℃ at the periphery. A lethal isotherm has been described as ranging from −20 to −70 ℃. A target temperature of −40 ℃ within the lethal isotherm is optimal to achieve cell death (12). Intracellular and extracellular ice crystal formation disrupts the cell membrane, with redistribution of solutes through freeze-thaw cycles, and causes tissue injury. Endothelial injury leads to small-vessel thrombosis and tissue ischemia.
The potential advantages of CA include the ability to see the ice ball forming under CT during the procedure. Multiple probes can be controlled individually, making it a good option if the location requires a high degree of precision. CA is also used in the treatment of larger tumors as multiple probes can be used to generate large ablation zones. It is thought that CA may be applicable for ablation of tumors near large airways, because the architecture of surrounding tissues tends to be preserved (13,14). CA also may cause less pain as compared with MWA and RFA, and patients may recover more quickly.
Limitations of CA include limited clinical data as compared with RFA, the longest setup time, and a time-consuming procedure due to the complexities of managing the gas and performing the ablation. Because some CA systems lack the natural cautery effect of heat-based ablation methods, CA is not preferred in patients with coagulopathies and bleeding tendencies. Bleeding and hemoptysis are known complications. One commercially available CA system has an internal heat-exchange function that can cauterize tissue to reduce bleeding.
Technique of performing the ablation
Anatomic considerations
CT imaging is typically used for planning percutaneous lung ablations. The needle electrode is most often introduced into the tumor percutaneously under CT guidance, and the tines of the probe are positioned in the lesion. Tumor position within the lobe and adjacent fissures and blood vessels and airways should be considered when positioning the patient. Peripheral lung nodules may be more difficult to target via a direct puncture that is perpendicular to the pleura, because the ablation applicator may not be well secured in the lung and could be displaced with breathing. To adequately secure the ablation needle in enough lung tissue for better anchorage, peripheral nodules are best approached tangentially. During the procedure, the applicator tip may end up closer to vital structures than originally planned, because lung volume will decrease during ablation, and this should be closely monitored. When a needle traverses a fissure, which consists of 2 layers of pleura, the risk of pneumothorax may be increased. The completeness of the fissure can be assessed on the CT scan and can be taken into consideration when planning the ablation (6,15).
Anesthesia and sedation
The type of anesthesia used depends on institutional and physician preference. Local anesthesia, typically with lidocaine is used. For prolonged anesthesia, bupivacaine is preferred, particularly if the pleura is involved. Although the choice of anesthesia is typically physician dependent, many physicians prefer general anesthesia because cardiopulmonary support can be provided during the procedure, which is important in patients with comorbidities or patients with limited cardiopulmonary reserve.
Ablation technique
Placement of the ablation applicators is similar between both the high and low temperature modalities, but there are specific technical details to follow for each ablation modality to optimize the ablation and avoid complications. RFA systems use single, clustered, or deployable multi-tined electrodes. Cluster electrodes often used ablate larger nodules, may be more difficult to place since the three electrodes can displace the nodule making it more difficult to puncture. Tined electrodes can also inadvertently displace the tumor rather than puncture it. This can typically be avoided with adequate preplanning and patient positioning, which is essential when the tumor is close to vital structures. In general we strive to obtain an adequate zone of ablation around the tumor for effective ablation.
Post-procedure care
Post-ablation, one of the authors (DED) prefers having patients placed in the decubitus position with the ablation side down. This limits lung motion and prevents blood and fluid from entering the contralateral lung. In a study of 373 consecutive patients treated with CT-guided biopsy, Drumm and colleagues reported that the rate of pneumothorax was significantly lower (P<0.001) in patients who were positioned with the side of biopsy down, as compared with patients positioned in the prone or supine position. (P<0.001) (16). The authors concluded that the positioning of the patient with the side of the biopsy down for percutaneous CT-guided lung biopsy had a lower incidence of pneumothorax as compared with the supine or prone position.
Complications involving the pleura or the lung can occur immediately after ablation and include pneumothorax, pleural effusion, hemothorax, and pleural thickening. Chest radiographs are obtained 1–3 hours post-procedure to exclude a significant hemothorax or pneumothorax.
Complications
Pneumothorax is the most reported complication after RFA or MWA. The incidence of pneumothorax is 30−60%, but not all patients with pneumothoraces require placement of a pigtail catheter (17). The threshold to place a pigtail pleural catheter for pneumothorax depends upon the size of the pneumothorax and severity of the underlying lung disease. MWA is also associated with a higher rate of bronchopleural fistula. Pleural hemorrhage is more common after CA, and drainage may be required for hemothorax and persistent or large pleural effusions (18). Other reported adverse events include pulmonary hemorrhage, pneumonia, or abscess formation (19). It is quite rare for complications to manifest more than 1 month after ablation.
Evaluation of response
After ablation, a consistent imaging protocol should be followed. This is essential to detect early complications, observe the natural evolution of the ablation zone, and detect recurrences. While follow-up protocols vary between institutions, imaging during post-ablation follow-up is essential and provides a framework for oncology practice. Imaging is obtained 3–4 weeks post-ablation, preferably with and without contrast (unless contraindicated). It is also important to recognize post-ablation imaging features to differentiate anticipated tumor regression versus local recurrence.
The protocol at the University of Pittsburgh is to follow the patients at 3-to-4-month intervals with clinical examinations, CT scans, and selectively with positron emission tomography (PET) scans. A modified RECIST (response evaluation criteria in solid tumors) criteria, which incorporates PET scans selectively in addition to CT scans, is utilized to evaluate response to treatment (4,9,20,21). Patients are evaluated for initial response, progression, and overall survival.
Results of treatment
RFA for stage I NSCLC
In medically inoperable (high-risk) patients with early-stage NSCLC, ablation has emerged as a safe and effective option. In a study using SEER and Medicare databases, Kwan and colleagues found no significant difference in overall survival in 75 patients with early-stage NSCLC treated with percutaneous thermal ablation as compared with 1,822 patients who underwent sublobar resection (22). Many reports of the results of RFA for stage I NSCLC have been published previously (21,23-37). Some of these results are summarized in Table 1 and below. As physicians have gained more experience and patient selection criteria have been established, improved long-term survival after RFA has been reported in recently published series. The American College of Chest Physicians included RFA in their most recent treatment recommendations for tumors measuring <3 cm (29).
Table 1
Study, author, year (ref) | No. of patients | Pathology | Duration of follow-up | Outcome results |
---|---|---|---|---|
Simon et al., 2007 (37) | 75 | Stage I NSCLC | Median: 20.5 months* | Estimated overall survival: 1, 2 and 5 years were 78%, 57% and 27%, respectively. Median survival: 29 months |
Lencioni et al., 2008 (33) | 13 | Stage I NSCLC | Mean: 15 months* | Overall survival at 2 years: 75% |
Ambrogi et al., 2011 (23) | 57 | Stage I NSCLC | Mean: 47 months | Median survival: 33.4 months. Estimated cancer-specific 1-, 3-, and 5-year survival: 89%, 59%, and 40% respectively |
Hiraki et al., 2007 (28) | 20 | Stage I NSCLC | Median: 21 months | Local progression: 35%. Estimated overall 1-, 2-, and 3-year survival: 90%, 84% and 74%, respectively |
Pennathur et al., 2007 (21) | 19 | Stage I NSCLC | Mean: 29 months | Overall survival at 1 year: 95%, 2 years: 68%. Median survival: NR. Local progression: 42%. Median time to local progression: 27 months |
Beland et al., 2010 (25) | 79 | NSCLC (stage I: 67 patients) | Mean: 16 months | Overall recurrence in 43%. Median disease-free survival: 23 months |
Lanuti et al., 2009 (30) | 31 | Stage I NSCLC | Median: 17.3 months | Estimated 2-year survival: 78%. Median survival: 30 months. Local progression: 31.5% |
Dupuy et al., 2015 (27) | 54 | Stage IA | Estimated overall survival at 1- and 2-year: 86.3% and 69.8% at two years, respectively. Estimated local tumor recurrence free rate: 68.9% at one year and 59.8% at two years | |
Palussière et al., 2018 (35) | 32 | Stage IA | The estimated overall survival was 91.67% at 1 year and 58.33% at 3 years |
*, follow-up duration was not reported specifically for patients with stage I NSCLC and includes follow up for all patients in this report. Adapted from Pennathur A et al. Image-guided radiofrequency ablation for the treatment of early-stage non-small cell lung neoplasm in high-risk patients. Semin Thorac Cardiovasc Surg 2010;22:53-8. Used with permission from Elsevier. NSCLC, non-small cell lung cancer; NR, not reached.
Clinical studies of RFA for stage I NSCLC
Liu and colleagues reported the results of RFA in 29 medically inoperable patients with stage I NSCLC. After RFA, overall 1-year survival was 90.5%, 2-year survival was 76.4%, and 3-year survival was 65.5% (34). The mean overall survival in these patients was 57 months. Local tumor progression occurred in 21% of patients with a follow-up duration of 25 months. Lee and colleagues reported their experience with RFA in 10 patients with stage I NSCLC, of which four patients were considered high-risk patients; 80% were alive at a mean follow-up of 14.8 months (28,32).
Hiraki and colleagues reported the outcomes in 20 patients (medically inoperable n=14; refused surgery n=6) with stage I NSCLC who were treated with RFA (28). Pneumothorax was the most common complication (57%). At a median follow-up of 21 months, 35% of patients (7 patients) had local progression. The median time to local progression was 9 months. The estimated overall survival was 90% at 1 year, 84% at 2 years, and 74% at 3 years. In a multicenter international study of RFA reported by Lencioni, thirteen of the 33 patients with NSCLC had stage I NSCLC (33). The primary endpoints in this study were technical success, safety, and response rates. Overall survival, cancer-specific survival, and quality of life were secondary endpoints. RFA was performed successfully from a technical standpoint in 99% of the patients. Among patients with NSCLC who were evaluated for response during a mean follow up of 15 months, 12.5% had an incomplete response or progression of disease. The estimated 2-year overall survival in patients with stage I NSCLC was 75%. Both the Lencioni study and the Hiraki study had a relatively short follow up period, and PET scans were not routinely used for response assessment in either study.
Simon and colleagues in a large study, presented the results of RFA in 75 patients with stage I NSCLC (75% stage IA and 25% stage IB) (37). The 30-day perioperative mortality rate after RFA was 3.9%, with 2.6% procedure-specific mortality. Median survival after RFA was 29 months with overall survival of 78% at 1 year, 57% at 2 years, and 27% at 5 years. Tumor size was significantly associated with progression-free survival, and 5-year local progression-free survival was higher in patients with tumors smaller than 3 cm than in patients with tumors larger than 3 cm.
Beland and colleagues reported the results of 79 patients with NSCLC who were treated with RFA (25). There were 54 patients with stage IA NSCLC and another 13 patients with stage IB NSCLC who were followed for a median of 16 months (range, 1–72 months) after RFA. In patients with recurrent disease (34 patients, 43%), progression was most often local (38%), with intrapulmonary (same lobe) recurrence in 18%, distal recurrence in 21%, and mixed (local and nodal) recurrence in 6%. Two years after RFA, the estimated local progression was 28%.
Outcomes after RFA for the treatment of stage I NSCLC in 31 patients over a four and half year period were reported in another study (30). Most of the patients had stage IA adenocarcinoma with a mean tumor size of 2 cm. In this series, 11 patients (35%) with comorbidities underwent RFA because they refused surgery. There was no perioperative mortality. During a median follow up of 17.3 months, 31.5% of patients experienced local recurrence or progression. The overall median survival was 30 months, and median disease-free survival was 25.5 months. Two years post-RFA, overall survival was 78% and estimated disease-free survival was 57%. Three years after RFA, overall survival was 47% and estimated disease-free survival was 39%. These investigators also evaluated pulmonary function at baseline and 3–6 months after RFA and noted that pulmonary function was preserved during early follow-up with no significant difference from baseline 3–6 months after RFA. Later, in an updated series, Lanuti reported the results of RFA in 45 patients with stage I NSCLC (31). At a median follow-up of 32 months, locoregional recurrence was reported in 38% of patients within a mean of 12 months from RFA. These authors reported locoregional recurrence in 80% of patients with tumors larger than 3 cm. After treatment for recurrence, the overall survival among patients who did or did not experience locoregional recurrence was similar (32% to 35%).
Ambrogi reported the results of RFA in 57 inoperable patients with stage I NSCLC (23). Two patients were treated for two separate lesions. There were total of 44 patients with stage IA lesions, and 15 with stage IB lesions. The mean size of the lesions was 2.6 cm. At a mean follow-up of 47 months, the median overall survival was 33.4 months and median cancer-specific survival was 41.4 months. Cancer-specific actuarial survival was 89% at 1 year, 59% at 3 years, and 40% at 5 years.
Promising outcomes have been observed in multicenter studies as well. Palussière and colleagues in a prospective multicenter study reported the results of RFA in 32 patients with stage IA NSCLC (35). The local control rate at 1-year was 84.4% (95% CI: 67.2–95.7%). and at 3-years was 81.3% (95% CI: 54.3–95.9%). The estimated overall survival was 91.7% at 1 year and 58.3% at 3 years. There was no significant change in the patients’ global health status or quality-of-life following RFA. Dupuy and colleagues published a prospective multicenter trial of RFA for stage IA NSCLC (27). Fifty-four patients with stage IA NSCLC were enrolled. The authors reported overall survival rate of 86.3% at 1 year and 69.8% at 2 years. The overall survival was 83% at 2 years when the tumor was <2.0 cm in size.
Results of ablation in medically inoperable, high-risk patients with stage I NSCLC treated with RFA under CT guidance at the University of Pittsburgh were previously published (21). One of the primary strengths of this study was the methodical and longer follow-up as compared with previous studies. A total of 19 patients (median age of 78 years) underwent RFA over a 3-year period. The mean follow-up was 29 months. An initial complete response (10.5%) or partial response (53%) was observed in 63.5% of patients with stable disease in 26% of patients. During follow-up, local progression of nodules occurred in 42% of patients and the median time to progression was 27 months. The procedure-related mortality was zero. The estimated probability of survival at 1 year was 95%, and the estimated probability of survival at 2 years was 68% (Figure 1). This experience indicated that RFA is safe in high-risk patients with stage I NSCLC and leads to reasonable outcomes in patients who are not fit for surgery. Few studies have examined results following combination therapy with percutaneous thermal ablation and radiation. Combination therapy has typically been used in patients with tumors that are difficult to treat with a single modality alone (38,39).
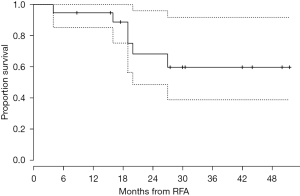
RFA for lung metastases
After the liver, the lungs are the most prominent organs for metastatic tumors. Surgery and local therapies, including SRS and image-guided thermal ablation, are available for patients with lung oligometastatic disease from various cancers. In patients with oligometastatic disease, surgical resection is the first-line method for treating pulmonary metastases. For patients with lung metastases ineligible for surgical treatment, radiotherapy and ablation therapy are most commonly used. With advances in radiotherapy (e.g., stereotactic radiosurgery), the efficacy of radiotherapy for lung metastases has significantly improved. However, due to dose restrictions, multiple radiation courses may not be feasible. Ablation therapy had been increasingly used for treatment of lung metastases because it is minimally invasive, and repeatable. The greatest advantage of ablation therapy is the ability to treat lung metastases and at the same time protect normal lung tissue.
Decision-making to use local therapies to treat metastases is complex, depends on clinical presentation and tumor histology, and should always engage a multidisciplinary team to discuss treatment options. It is essential to avoid overtreatment. Local treatment for a single metastasis or limited metastatic disease in a patient with well-controlled disease is agreed on by most experts, but most will refrain from local treatment of rapidly evolving metastatic disease at multiple sites. The challenge is determining appropriate treatments for patients between these 2 extremes, for whom the potential benefit of disease control on overall survival must be evaluated.
Clinical studies of RFA for lung metastases
Surgical resection is beneficial in selected patients with pulmonary metastases. The International Registry for Lung Metastases reported the results of 5,206 patients who were treated with surgical resection for pulmonary metastases (40). After complete resection, the estimated 5-year survival was 36%, and the median survival was 35 months. Recurrences occurred in 53% of patients, and the median time to recurrence was 10 months. The criteria used for selection of patients for surgical resection include: Complete control of primary tumor, no extra-thoracic disease, a long disease-free interval, and limited pulmonary metastases.
Complete resection of pulmonary metastases is an important prognostic factor. The results of RFA for the treatment of pulmonary metastases at the University of Pittsburgh Medical Center (UPMC), either as a single modality or in combination with surgical resection, have been published (41). Twenty-two patients with metastatic lung disease underwent RFA. RFA was the sole treatment in 17 patients (77%) and was combined with surgical resection in 5 (23%). The median survival after a mean follow up of 27 was 29 months. Tumor size was significantly associated with both recurrence and survival (P<0.05). The median survival for patients with a lesion diameter of 3 cm or less was 39.1 vs. 7.9 months for patients with lesion diameter exceeding 3 cm (P=0.002). Similarly, progression-free survival was better in patients with a lesion smaller than 3 cm. In an updated series of 100 patients, the results of RFA for the treatment of limited pulmonary metastases in 29 patients at the University of Pittsburgh have been reported (42). The probability of 2-year overall survival for metastatic cancer patients was 41%. Yan and colleagues reported the results of RFA for the treatment of pulmonary metastases in 55 patients with primary colorectal neoplasms (43,44). The median survival in these patients was 33 months, and the estimated 2-year survival was 64%.
The largest report of RFA for lung metastases included 566 patients treated for 1,037 lung metastases. Most patients had 1 or 2 lung metastases (53% with 1 metastasis and 25% with 2), but some were treated for up to 8 lung metastases (45). The median follow-up was 35.5 months. Median overall survival was 62 months and was influenced by the location of the primary malignancy, the disease-free interval, and the size and number of metastases. Local tumor progression at the site of RFA was associated with poor overall survival. Approximately 90% of the patients treated had a complete response for at least 1 year, and the high local control rates observed were achieved in part by applying strict selection criteria informed by extensive pre-RFA imaging. In fact, the low rate of local tumor progression observed was similar to rates of progression reported after surgical resection of lung metastases (33).
RFA for recurrent lung cancer
Despite complete resection or definitive chemoradiation of lung cancer, 25% to 70% of patients have disease recurrence (46,47). In patients who have previously undergone successful lung resection for cancer, recurrent disease is the most common cause of cancer-related mortality (48). The reported results of reoperation for recurrence have not been encouraging, with 2-year survival of 23% and 5-year survival of 15% (47-49). In the event of recurrence after previous lung resection, pulmonary function may be compromised. In patients with recurrent disease after previous chemoradiation, the ability to treat with radiation may be limited by cumulative dose restraints. RFA may offer an alternative treatment option in selected patients with recurrent lung cancer because it can be repeated and is not limited by the cumulative dose constraints.
The results of RFA in 25 patients who developed recurrent NSCLC were reported by authors at UPMC (42). Their median overall survival was 33 months. The estimated 2-year overall survival for these patients with recurrent lung neoplasm was 55%. Additionally, the results of a systematic review of 6 studies with a total of 226 patients with recurrent NSCLC who were treated with RFA were presented (50,51). The estimated 1-year survival rates reported ranged from 20% to 97.7% while 1-year tumor recurrence rates ranged from 37.7% to 43.2%. The follow-up was limited, however, and one of the main conclusions was that longer follow-up with more patients is needed.
Conclusions
Percutaneous image-guided ablation of lung neoplasm is a therapeutic option for carefully selected patients with primary lung cancer, recurrent lung cancer, and limited pulmonary metastases. There are many potential benefits to ablative therapy. Ablation requires a shorter hospital stay and recovery time as compared with traditional surgical procedures, may be used to treat tumors when surgery or radiation are not options, and can relieve pain or other debilitating symptoms. There are several factors that warrant further investigation including optimal patient selection criteria and measures to decrease local recurrence of the tumor.
While surgery remains the standard for early-stage resectable lung cancer, emerging technologies, such as RFA, play a role in high-risk and medically inoperable patients. Image-guided RFA is feasible and safe in high-risk patients with lung neoplasm, with reasonable results in this patient population. Since it allows for a potential shorter recovery time in medically inoperable high-risk patients and is repeatable, image-guided thermal ablation of lung neoplasm in selected patients is gaining acceptance in the oncology community. Lung ablation may become a more feasible and effective treatment as providers gain more experience and the technology advances. New technologies should continue to be evaluated, such as ablative techniques, to add to the armamentarium for the treatment of lung neoplasm.
Panel discussion
What is the surgical approach for selection of patients with early-stage NSCLC?
Pennathur: In our previous study, we described the selection of patients for RFA for the treatment of stage I NSCLC (21). Patients were staged routinely with chest CT scanning, and most patients also underwent a PET scan. Patients with enlarged mediastinal lymph nodes are staged with mediastinoscopy, and more recently with endobronchial ultrasound (EBUS). Our inclusion criteria for RFA in the treatment of patients with stage I NSCLC for that study were: (I) patients who were considered medically inoperable and (II) presence of a target tumor of ≤4 cm. In addition, patients who refused an operation were offered RFA if the tumor was peripherally located and <4 cm. Patients with central tumors were excluded from the study. All patients are evaluated by a thoracic surgeon to determine inoperability and suitability for RFA.
Dupuy: Many of the patients we see have multiple comorbidities. If we are treating them for an early-stage lung cancer, we require fluorodeoxyglucose (FDG) PET-CT to help stage the patient. If there is any suspicious nodal activity, we would suggest biopsy with EBUS techniques or mediastinoscopy. We assess the patient’s comorbidities including the severity of underlying emphysema or pulmonary fibrosis. Pulmonary function tests are usually available, and if not, we may obtain them if our assessment suggests severe underlying respiratory compromise that may make the procedure too risky or may not provide meaningful overall survival. If we feel the patient is unlikely to live longer than 1 year with their current comorbidities, we would not recommend treating their early-stage lung cancer.
What is the technique followed for the treatment of lung lesions by RFA? Any tips on decreasing the incidence of pneumothorax and decreasing the recurrence of tumor in treated nodules? Any other technical details or modifications based on the location of the tumor?
Pennathur: The principles of RFA are similar using various devices. At UPMC, we use a standard percutaneous CT-guided approach that we have described previously (4,21). Electrosurgical dispersive pads are applied to the patient’s thighs and plugged into the return electrode socket of the radiofrequency generator. The radiofrequency generator and needle electrodes are set up in accordance with the manufacturer’s instructions for use (RF3000; Boston Scientific Corp., Boston, MA, USA, or the RITA Starburst XL Electrosurgical Device; Angiodynamics, Latham, NY, USA). Based on the size of the target tumor, a multi-tined expandable array may be deployed. The electrode is repositioned as many times as necessary to encompass the target tissue and a 0.5 to 1 cm rim of non-diseased pulmonary tissue to ensure an adequate tumor margin.
Pneumothorax is the most frequent complication after this procedure, particularly when we biopsy and place fiducials in addition to RFA treatment. The trajectory of the probe is determined, and we select a window under CT guidance where the least amount of lung parenchyma is traversed during placement to decrease chances of a pneumothorax. This trajectory is verified, and the probe is placed. We generally utilize RFA in patients with compromised pulmonary function, with severe underlying lung disease. We have a low threshold to place a pleural tube to decompress the pneumothorax is these patients, typically with significant underlying lung disease.
Dupuy: Tumors within 1 cm of the heart may require an artificial pneumothorax for safety. Tumors close to the esophagus can be separated with saline hydrodissection in many instances. I treat many pleural-based tumors, and I use lidocaine and bupivacaine hydrodissection to reduce pain intraprocedurally and prevent thermal injury to the intercostal nerves. When we use MWA, we have to make sure that the trajectory includes a longer segment of lung tissue when we are using higher frequency (2,450 MHz) devices. This is important because temperatures with these devices can emit steam back along the needle track, which may increase the risk of bronchopleural fistula. With lower frequency devices (e.g., 915 MHz) this is less of an issue, similar to RFA. In my experience, using a bolus of extrapleural lidocaine or bupivacaine can not only provide improved pain control during the procedure, but also hydrostatic pressure on the puncture at the end of the procedure that may reduce pneumothorax.
To decrease the risk of local recurrence, I try to place the devices medial to the lesion closer to potential heat sinks. I monitor the ground-glass density that develops around the treatment site and try to create overlapping ablations to generate a 1-cm-thick ground-glass density at the periphery on the non-pleural-based regions of the target.
Can RFA be combined with surgery or other modalities to treat lung neoplasm?
Pennathur: Yes, we use RFA in combination with other modalities of treatment. For example, in patients in whom we feel that there is a higher risk of recurrence due to size of the lesion or other technical factors such as smaller margins of ablation, we place fiducials at the same time for future treatment with SRS/SBRT if there is progression of the lesion. In patients with pulmonary metastases, we have utilized surgical resection in combination with RFA as a parenchymal-sparing method, as described above (41).
Dupuy: In patients with larger lung lesions poorly suited for SBRT, where more durable local control is desired (e.g., chest wall invasion), thermal ablation with CA or MWA can be done in conjunction with hyperfractionated radiotherapy techniques. In patients with oligonodular metastatic disease, thermal ablation can be performed in areas where thermal sinks adjacent to pulmonary vessels are not a concern (less than 4 mm in diameter). SBRT can be used in areas adjacent to vessels larger than 4 mm in diameter.
Perspective statement
Pennathur: Surgical resection is the standard of care for early-stage NSCLC. Newer minimally invasive techniques, such as VATS resection, offer a lower morbidity option, however, with an aging population, and patients with comorbidities, a significant proportion of patients are not candidates for surgical resection (3). Ablative modalities, such as RFA, are applicable in this high-risk group of patients. One of the drawbacks of RFA is a higher local recurrence rate when compared with surgery and SRS/SBRT. Newer localization techniques, which are robot guided, as well as percutaneous navigation systems may assist in deployment of the RFA probe in the lesion. In addition, computational models of isotherms forming a contour map may help identify tissue at risk for under-treatment and thus decrease the local recurrence rates (52). In this paper, we have described the results primarily of CT-guided RFA. In the future, RFA delivered with navigational bronchoscopy guidance may be possible (53,54). Thoracic surgeons should continue to evaluate new technologies, such as ablative techniques, and add these to their armamentarium in the treatment of lung neoplasm.
Dupuy: During local treatment of lung tumors, induction of an anti-tumoral immune response has been observed in patients with different tumor entities during thermal ablation in addition to cell necrosis (55). This was initially noted, after observation of regression of distant untreated tumors in patients treated with thermal ablation. Within a few hours to days following ablation, necrotic cells released their contents in the extracellular space and triggered an immune response. Heat shock proteins are elevated after tumor ablation and seem to be of importance for the activation of anti-tumor T cells. Enhancement of this effect with additional immunomodulation can be a future treatment option for patients (55).
Acknowledgments
Funding: This study was supported in part by the Sampson Family Endowed Chair in Thoracic Surgical Oncology at the University of Pittsburgh to AP.
Footnote
Provenance and Peer Review: This article was commissioned by the Guest Editors (Scott Swanson, Daniel Dolan) for the series “How to Evaluate, Diagnose and Treat Small Lung Nodules” published in Current Challenges in Thoracic Surgery. The article has undergone external peer review.
Conflicts of Interest: All authors have completed the ICMJE uniform disclosure form (available at https://ccts.amegroups.com/article/view/10.21037/ccts-22-7/coif). The series “How to Evaluate, Diagnose and Treat Small Lung Nodules” was commissioned by the editorial office without any funding or sponsorship. DED receives consulting fees from Varian, royalties from Springer-Verlag, UptoDate and fees related to expert witness testimony. DED has issued and pending patents and stock options related to Theromics Inc and Lozeta. DED is on the Annual Meeting Committee of The Society of Interventional Oncology. The authors have no other conflicts of interest to declare.
Ethical Statement: The authors are accountable for all aspects of the work in ensuring that questions related to the accuracy or integrity of any part of the work are appropriately investigated and resolved.
Open Access Statement: This is an Open Access article distributed in accordance with the Creative Commons Attribution-NonCommercial-NoDerivs 4.0 International License (CC BY-NC-ND 4.0), which permits the non-commercial replication and distribution of the article with the strict proviso that no changes or edits are made and the original work is properly cited (including links to both the formal publication through the relevant DOI and the license). See: https://creativecommons.org/licenses/by-nc-nd/4.0/.
References
- Siegel RL, Miller KD, Fuchs HE, et al. Cancer statistics, 2022. CA Cancer J Clin 2022;72:7-33. [Crossref] [PubMed]
- Mazzone P. Preoperative evaluation of the lung resection candidate. Cleve Clin J Med 2012;79 Electronic Suppl 1:eS17-22.
- Pennathur A, Brunelli A, Criner GJ, et al. Definition and assessment of high risk in patients considered for lobectomy for stage I non-small cell lung cancer: The American Association for Thoracic Surgery expert panel consensus document. J Thorac Cardiovasc Surg 2021;162:1605-1618.e6. [Crossref] [PubMed]
- Pennathur A, Abbas G, Schuchert M, et al. Radiofrequency ablation for the treatment of lung neoplasm. Expert Rev Med Devices 2008;5:613-21. [Crossref] [PubMed]
- Dupuy DE, Zagoria RJ, Akerley W, et al. Percutaneous radiofrequency ablation of malignancies in the lung. AJR Am J Roentgenol 2000;174:57-9. [Crossref] [PubMed]
- Abtin F, De Baere T, Dupuy DE, et al. Updates on Current Role and Practice of Lung Ablation. J Thorac Imaging 2019;34:266-77. [Crossref] [PubMed]
- Alexander ES, Machan JT, Ng T, et al. Cost and effectiveness of radiofrequency ablation versus limited surgical resection for stage I non-small-cell lung cancer in elderly patients: is less more? J Vasc Interv Radiol 2013;24:476-82. [Crossref] [PubMed]
- Kwan SW, Mortell KE, Hippe DS, et al. An economic analysis of sublobar resection versus thermal ablation for early-stage non-small-cell lung cancer. J Vasc Interv Radiol 2014;25:1558-64; quiz 1565. [Crossref] [PubMed]
- Sharma A, Abtin F, Shepard JA. Image-guided ablative therapies for lung cancer. Radiol Clin North Am 2012;50:975-99. [Crossref] [PubMed]
- Brace CL. Radiofrequency and microwave ablation of the liver, lung, kidney, and bone: what are the differences? Curr Probl Diagn Radiol 2009;38:135-43. [Crossref] [PubMed]
- Al-Hakim RA, Abtin FG, Genshaft SJ, et al. Defining New Metrics in Microwave Ablation of Pulmonary Tumors: Ablation Work and Ablation Resistance Score. J Vasc Interv Radiol 2016;27:1380-6. [Crossref] [PubMed]
- Gage AA, Baust J. Mechanisms of tissue injury in cryosurgery. Cryobiology 1998;37:171-86. [Crossref] [PubMed]
- Abtin F, Quirk MT, Suh RD, et al. Percutaneous Cryoablation for the Treatment of Recurrent Malignant Pleural Mesothelioma: Safety, Early-Term Efficacy, and Predictors of Local Recurrence. J Vasc Interv Radiol 2017;28:213-21. [Crossref] [PubMed]
- Abtin F, Suh RD, Nasehi L, et al. Percutaneous cryoablation for the treatment of recurrent thymoma: preliminary safety and efficacy. J Vasc Interv Radiol 2015;26:709-14. [Crossref] [PubMed]
- Aziz A, Ashizawa K, Nagaoki K, et al. High resolution CT anatomy of the pulmonary fissures. J Thorac Imaging 2004;19:186-91. [Crossref] [PubMed]
- Drumm O, Joyce EA, de Blacam C, et al. CT-guided Lung Biopsy: Effect of Biopsy-side Down Position on Pneumothorax and Chest Tube Placement. Radiology 2019;292:190-6. [Crossref] [PubMed]
- Kawamura M, Izumi Y, Tsukada N, et al. Percutaneous cryoablation of small pulmonary malignant tumors under computed tomographic guidance with local anesthesia for nonsurgical candidates. J Thorac Cardiovasc Surg 2006;131:1007-13. [Crossref] [PubMed]
- Littrup PJ, Mody A, Sparschu R, et al. Prostatic cryotherapy: ultrasonographic and pathologic correlation in the canine model. Urology 1994;44:175-83; discussion 183-4. [Crossref] [PubMed]
- Chan VO, McDermott S, Malone DE, et al. Percutaneous radiofrequency ablation of lung tumors: evaluation of the literature using evidence-based techniques. J Thorac Imaging 2011;26:18-26. [Crossref] [PubMed]
- Pennathur A, Abbas G, Landreneau RJ, et al. Radiofrequency ablation for the treatment of stage I non-small cell lung neoplasm. Semin Thorac Cardiovasc Surg 2008;20:279-84. [Crossref] [PubMed]
- Pennathur A, Luketich JD, Abbas G, et al. Radiofrequency ablation for the treatment of stage I non-small cell lung cancer in high-risk patients. J Thorac Cardiovasc Surg 2007;134:857-64. [Crossref] [PubMed]
- Kwan SW, Mortell KE, Talenfeld AD, et al. Thermal ablation matches sublobar resection outcomes in older patients with early-stage non-small cell lung cancer. J Vasc Interv Radiol 2014;25:1-9.e1. [Crossref] [PubMed]
- Ambrogi MC, Fanucchi O, Cioni R, et al. Long-term results of radiofrequency ablation treatment of stage I non-small cell lung cancer: a prospective intention-to-treat study. J Thorac Oncol 2011;6:2044-51. [Crossref] [PubMed]
- Ambrogi MC, Lucchi M, Dini P, et al. Percutaneous radiofrequency ablation of lung tumours: results in the mid-term. Eur J Cardiothorac Surg 2006;30:177-83. [Crossref] [PubMed]
- Beland MD, Wasser EJ, Mayo-Smith WW, et al. Primary non-small cell lung cancer: review of frequency, location, and time of recurrence after radiofrequency ablation. Radiology 2010;254:301-7. [Crossref] [PubMed]
- Donington J, Ferguson M, Mazzone P, et al. American College of Chest Physicians and Society of Thoracic Surgeons consensus statement for evaluation and management for high-risk patients with stage I non-small cell lung cancer. Chest 2012;142:1620-35. [Crossref] [PubMed]
- Dupuy DE, Fernando HC, Hillman S, et al. Radiofrequency ablation of stage IA non-small cell lung cancer in medically inoperable patients: Results from the American College of Surgeons Oncology Group Z4033 (Alliance) trial. Cancer 2015;121:3491-8. [Crossref] [PubMed]
- Hiraki T, Gobara H, Iishi T, et al. Percutaneous radiofrequency ablation for clinical stage I non-small cell lung cancer: results in 20 nonsurgical candidates. J Thorac Cardiovasc Surg 2007;134:1306-12. [Crossref] [PubMed]
- Howington JA, Blum MG, Chang AC, et al. Treatment of stage I and II non-small cell lung cancer: Diagnosis and management of lung cancer, 3rd ed: American College of Chest Physicians evidence-based clinical practice guidelines. Chest 2013;143:e278S-313S.
- Lanuti M, Sharma A, Digumarthy SR, et al. Radiofrequency ablation for treatment of medically inoperable stage I non-small cell lung cancer. J Thorac Cardiovasc Surg 2009;137:160-6. [Crossref] [PubMed]
- Lanuti M, Sharma A, Willers H, et al. Radiofrequency ablation for stage I non-small cell lung cancer: management of locoregional recurrence. Ann Thorac Surg 2012;93:921-7; discussion 927-88. [Crossref] [PubMed]
- Lee JM, Jin GY, Goldberg SN, et al. Percutaneous radiofrequency ablation for inoperable non-small cell lung cancer and metastases: preliminary report. Radiology 2004;230:125-34. [Crossref] [PubMed]
- Lencioni R, Crocetti L, Cioni R, et al. Response to radiofrequency ablation of pulmonary tumours: a prospective, intention-to-treat, multicentre clinical trial (the RAPTURE study). Lancet Oncol 2008;9:621-8. [Crossref] [PubMed]
- Liu B, Liu L, Hu M, et al. Percutaneous radiofrequency ablation for medically inoperable patients with clinical stage I non-small cell lung cancer. Thorac Cancer 2015;6:327-33. [Crossref] [PubMed]
- Palussière J, Chomy F, Savina M, et al. Radiofrequency ablation of stage IA non-small cell lung cancer in patients ineligible for surgery: results of a prospective multicenter phase II trial. J Cardiothorac Surg 2018;13:91. [Crossref] [PubMed]
- Pennathur A, Abbas G, Schuchert MJ, et al. Image-guided radiofrequency ablation for the treatment of early-stage non-small cell lung neoplasm in high-risk patients. Semin Thorac Cardiovasc Surg 2010;22:53-8. [Crossref] [PubMed]
- Simon CJ, Dupuy DE, DiPetrillo TA, et al. Pulmonary radiofrequency ablation: long-term safety and efficacy in 153 patients. Radiology 2007;243:268-75. [Crossref] [PubMed]
- Chan MD, Dupuy DE, Mayo-Smith WW, et al. Combined radiofrequency ablation and high-dose rate brachytherapy for early-stage non-small-cell lung cancer. Brachytherapy 2011;10:253-9. [Crossref] [PubMed]
- Sandler KA, Abtin F, Suh R, et al. A Prospective Phase 2 Study Evaluating Safety and Efficacy of Combining Stereotactic Body Radiation Therapy With Heat-based Ablation for Centrally Located Lung Tumors. Int J Radiat Oncol Biol Phys 2018;101:564-73. [Crossref] [PubMed]
- Pastorino U, Buyse M, Friedel G, et al. Long-term results of lung metastasectomy: prognostic analyses based on 5206 cases. J Thorac Cardiovasc Surg 1997;113:37-49. [Crossref] [PubMed]
- Pennathur A, Abbas G, Qureshi I, et al. Radiofrequency ablation for the treatment of pulmonary metastases. Ann Thorac Surg 2009;87:1030-6; discussion 1036-9. [Crossref] [PubMed]
- Pennathur A, Abbas G, Gooding WE, et al. Image-guided radiofrequency ablation of lung neoplasm in 100 consecutive patients by a thoracic surgical service. Ann Thorac Surg 2009;88:1601-6; discussion 1607-8. [Crossref] [PubMed]
- Yan TD, King J, Sjarif A, et al. Treatment failure after percutaneous radiofrequency ablation for nonsurgical candidates with pulmonary metastases from colorectal carcinoma. Ann Surg Oncol 2007;14:1718-26. [Crossref] [PubMed]
- Yan TD, King J, Sjarif A, et al. Percutaneous radiofrequency ablation of pulmonary metastases from colorectal carcinoma: prognostic determinants for survival. Ann Surg Oncol 2006;13:1529-37. [Crossref] [PubMed]
- de Baère T, Aupérin A, Deschamps F, et al. Radiofrequency ablation is a valid treatment option for lung metastases: experience in 566 patients with 1037 metastases. Ann Oncol 2015;26:987-91. [Crossref] [PubMed]
- Pisters KM, Le Chevalier T. Adjuvant chemotherapy in completely resected non-small-cell lung cancer. J Clin Oncol 2005;23:3270-8. [Crossref] [PubMed]
- Zimmermann FB, Molls M, Jeremic B. Treatment of recurrent disease in lung cancer. Semin Surg Oncol 2003;21:122-7. [Crossref] [PubMed]
- Hung JJ, Hsu WH, Hsieh CC, et al. Post-recurrence survival in completely resected stage I non-small cell lung cancer with local recurrence. Thorax 2009;64:192-6. [Crossref] [PubMed]
- Pairolero PC, Williams DE, Bergstralh EJ, et al. Postsurgical stage I bronchogenic carcinoma: morbid implications of recurrent disease. Ann Thorac Surg 1984;38:331-8. [Crossref] [PubMed]
- Crentsil V, Luketich JD, Zhang D, et al. Radiofrequency Ablation for the Treatment of Recurrent Non-Small Cell Lung Neoplasm (NSCLC). Association of Veterans Affairs Surgeons 2021 "Virtual" Annual Meeting; Virtual; April 25, 2021.
- Crentsil VC, Zhang D, Luketich JD, et al. A Systematic Review of Radiofrequency Ablation for the Treatment of Recurrent NSCLC. American Federation for Medical Research - Eastern Regional Meeting; Virtual; April 2, 2021;
- Wood BJ, Locklin JK, Viswanathan A, et al. Technologies for guidance of radiofrequency ablation in the multimodality interventional suite of the future. J Vasc Interv Radiol 2007;18:9-24. [Crossref] [PubMed]
- Tanabe T, Koizumi T, Tsushima K, et al. Comparative study of three different catheters for CT imaging-bronchoscopy-guided radiofrequency ablation as a potential and novel interventional therapy for lung cancer. Chest 2010;137:890-7. [Crossref] [PubMed]
- Koizumi T, Tsushima K, Tanabe T, et al. Bronchoscopy-Guided Cooled Radiofrequency Ablation as a Novel Intervention Therapy for Peripheral Lung Cancer. Respiration 2015;90:47-55. [Crossref] [PubMed]
- Lyu N, Kong Y, Li X, et al. Ablation Reboots the Response in Advanced Hepatocellular Carcinoma With Stable or Atypical Response During PD-1 Therapy: A Proof-of-Concept Study. Front Oncol 2020;10:580241. [Crossref] [PubMed]
Cite this article as: Dupuy DE, Zuromski J, Pennathur A. Ablation for treating small pulmonary nodules: radiologic and surgical approach and perspectives. Curr Chall Thorac Surg 2022;4:40.