Lung cancer screening—contemporary issues: a narrative review
Introduction
Background
Lung cancer is the second most diagnosed cancer and the leading cause of cancer deaths globally with 1.8 million annual deaths (1). This high mortality rate is largely due to late diagnosis. Reduction in lung cancer mortality by screening with low-dose computed tomography (LDCT) versus no screening or chest X-ray in high-risk individuals who have ever smoked has been firmly established by meta-analyses and randomized controlled trials that included the pivotal National Lung Screening Trial (NLST) and the Dutch-Belgian NELSON trial (2-6). Lung cancer CT screening programs have been implemented at the country or regional level in various settings including the United States, Canada, South Korea, Poland, China, Croatia, Czech Republic, Taiwan and the United Arab Emirates. A further six countries—the United Kingdom, Australia, Italy, Slovakia, Romania and Kazakhstan, have formal commitment to implement LDCT screening with a number of other countries in the planning process (7). Successful implementation of an effective, high quality lung cancer screening program that can improve lung cancer outcomes requires attention to several key areas. The purpose of this review is to address the contemporary issues relevant to health professionals and policymakers regarding lung cancer screening and the optimal approach to integrating smoking cessation with screening.
Rationale and knowledge gaps
Unlike other cancer screening programs such as mammography screening for breast cancer that are based on age criteria, only those with sufficient risk for lung cancer that outweigh the potential harms of screening are screened. For a screening program to have substantial public health impact, major issues such as the proportion of high-risk individuals potentially covered by the screening eligibility criteria, workforce and technical capacity, equitable access to screening services, screening uptake, efficiency and safety of the screening management protocol and optimal smoking cessation intervention for participants who are still smoking need to be addressed.
Objective
The purpose of this narrative review is to discuss the contemporary issues in lung cancer screening relevant to health professionals, and policy development in the implementation of an effective lung screening program at the population level and the optimal approach to integrating smoking cessation into screening programs. We present this article in accordance with the Narrative Review reporting checklist (available at https://ccts.amegroups.com/article/view/10.21037/ccts-23-3/rc).
Methods
An English language literature search of PubMed, Cochrane Library and Web of Science was conducted using the keywords related to lung cancer screening [lung cancer, lung cancer screening, early detection, tobacco smoking, smoking cessation, disparities in lung cancer, biomarkers, cost-effectiveness]. The date of the last search was 6th April 2023 (Table 1).
Table 1
Items | Specification |
---|---|
Date of search | 04/06/2023 |
Databases and other sources searched | PubMed, Cochrane Library, Web of Science |
Search terms used | Lung cancer; lung cancer screening; early detection, tobacco smoking, smoking cessation; disparities in lung cancer; biomarkers; cost-effectiveness |
Timeframe | 2000 to 2023 |
Inclusion and exclusion criteria | Inclusion: English language literature, clinical trials, screening guidelines or statements by professional societies or regulatory agencies |
Exclusion: opinion articles, non-scientific or regulatory documents | |
Selection process | By authors following review and discussion |
Contemporary issues
Screening eligibility
Unlike other cancer screenings such as cervix, breast and colorectal cancer screening, lung screening only benefits those with sufficient risk of lung cancer and not everyone within a certain age group. For example, in the NLST, individuals who had a 6-year lung cancer risk <0.64%, had no significant reduction in lung cancer deaths in the LDCT group compared to the chest X-ray group (8) (Figure 1).
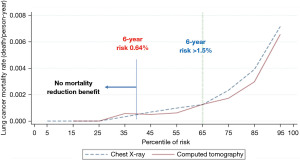
There are two major approaches to select high-risk ever smokers for LDCT screening. The first is categorical age (age 50/55 to 74/77/80 years), smoking (15/18.8/20/30 pack-years) and time since quitting (10/15 years for former smokers) that has been used by NELSON and NLST, and recommended by the US Medicare and Medicaid Services, the US Preventive Services Task Force (USPSTF 2013 and 2021) and the 2016 Canadian Task Force on Preventive Health Care as screening criteria (5,6,9-12). A second approach is to use risk prediction models that are based on incidence lung cancer risk or risk of lung cancer death (13). In contrast to using limited categorical risk criteria, accurate lung cancer risk prediction models use additional predictors and quantify risk by modeling continuous predictors. Over 20 lung cancer risk prediction models have been proposed (14). Several models have been identified as being accurate and possibly suitable for guiding selection of individuals for lung cancer screening and they include the Bach, LCDRAT, LLP and PLCOm2012 models (13,15-17). To date, the PLCOm2012 is the lung cancer risk prediction model that has been most validated by different research teams in multiple countries around the world, including the United States, Germany, Australia, the UK, Canada and Brazil (14-16,18-26). It is being used in Canada and the UK. The PLCOm2012 model has the following predictors: age, race/ethnicity, education (estimator of socioeconomic circumstance), body mass index, history of chronic obstructive pulmonary disease (COPD), personal history of cancer, family history of lung cancer, smoking status (current vs. former), smoking intensity (cigarettes per day), smoking duration and smoking quit-years in former smokers. In retrospective and cost-effectiveness analyses, Canada yields more life years gained, has smaller number needed to screen to avert one lung cancer death and is more cost-effective compared to USPSTF 2013 categorical criteria (8,13,22,27-32). A recent cost-effectiveness analysis compared risk-based strategies with the USPSTF 2013 and 2021 recommendations showed that PLCOm2012 was cost-effective down to a risk threshold of 1.2%/6 years with an incremental cost-effectiveness being more cost-effective than the USPSTF 2013 or 2021 recommendations, which were both under the analysis’ efficiency frontier and strongly dominated by other strategies that result in more quality-adjusted life years (QALYs) for similar costs, while having a similar level of screening coverage (person ever screened 21.7% with PLCOm2012 1.2%/6 years versus 22.6% with USPSTF) (33). Head-to-head comparison between USPSTF 2013 and PLCOm2012 showed the PLCOm2012 prediction tool was 15.8% more sensitive in identifying individuals with lung cancer screening the same number of participants (34). The positive predictive value was also significantly higher (4.0% vs. 3.4%, P=0.01). Of those deemed ineligible for lung screening based on PLCOm2012 or USPSTF criteria, lung cancer developed in 0.5% vs. 0.85%, respectively (P<0.001). Females typically accumulate fewer pack-years than male smokers (35). As a result, age and pack-years criteria underestimates lung cancer risk in females. Compared to non-indigenous people, indigenous people have a higher risk of lung cancer despite smoking less tobacco (36). PLCOm2012 race model removes race/ethnicity disparity and reduce sex disparity more than USPSTF 2021 screening criteria (34,35,37). The USPSTF 2021 criteria also exclude those who have stopped smoking for more than 15 years. A recent meta-analysis showed the reducible relative risk after smoking cessation only marginally declines after 15 years from 26.7% [95% confidence interval (CI): 20.2–34.3%] to 19.7% (95% CI: 13.3–26.4%) at 20 years (38). The duration of smoking cessation is not an exclusion criterion in PLCOm2012. In people who have stopped smoking, LDCT screening is one of the best options to reduce the risk of dying from lung cancer (39). An additional advantage of using the PLCOm2012 to determine screening eligibility is that screening can be prioritized according to individual risk scores, with the highest scoring individuals being offered screening first. This aspect is particularly beneficial when LDCT resources are limited, such as during the COVID-19 pandemic (40).
Since risk prediction models developed in a predominant North American-European population need to be validated in an Asian population to inform policy makers to design lung cancer screening programs, methodologies for recalibrating or adapting risk models were developed by synthesizing data sources without large prospective cohorts with long term follow-up. The PLCOm2012 was recalibrated for a Taiwanese population with high predictive performance and calibration (41). Prospective validation of the model is needed.
Lung cancer deaths related to tobacco smoking are projected to decrease in the next 25 years but the number and proportion of lung cancers in people who have never smoked will continue to increase (42). In some East Asian countries and regions, the incidence of lung adenocarcinoma has been increasing over time, despite a steady decline in male smoking since the 1990’s and a constant low smoking rate among females (43). The TALENT trial in Taiwan recruited people who have never smoked and had risk factors such as a family history of lung cancer, secondhand tobacco smoke exposure, chronic lung diseases such as tuberculosis or chronic obstructive pulmonary disease, high cooking index and cooking in poorly ventilated spaces (44). Among 12,011 participants, the first screening round revealed a 2.1% detection rate of stage I or higher lung cancers (44), a rate that is higher than in NLST (5) and NELSON (6). A prior 8-year population-based screening study was conducted in Hitachi City, Japan among individuals 50 to 74 years of age. In 17,935 participants who underwent LDCT and 15,548 controls who underwent one or more chest radiographs, 54% and 66% in the LDCT and chest-ray group respectively, had never smoked (45). Using regional cancer registry data, LDCT screening increased the lung cancer detection rate [hazard ratio (HR): 1.79] and decreased the lung cancer mortality rate (HR: 0.41) among the never smokers during year 4 to 8 of the screening compared to the first 4 years (45). However, a study in Taiwan showed a 6-fold increase in the incidence of stages 0–I lung cancer from 2004 to 2018 with no significant change in the incidence of stages II–IV lung cancer (46). The Shanghai Cancer Surveillance Study in China from 2002 to 2017 also showed a sharp rise in early-stage adenocarcinoma, particularly among young women with no significant decline in late-stage disease and mortality (47). These studies raised concern about overdiagnosis especially in younger females without identifiable lung cancer risk factor(s) (46,47). However, these studies did not discuss LDCT screening as a general health examination that includes younger individuals with no known lung cancer risk factor is different than screening based on risk factors such as family history of lung cancer, genetics, outdoor and indoor exposures (48). In addition, overdiagnosis related to the relatively high proportion of adenocarcinomas in-situ (stage 0) can be reduced by improvement in our understanding of the molecular biology of non-solid (ground-glass) nodules (GGNs) and refinement of the lung nodule management protocol (48).
Several lung cancer risk assessment tools for people who have never smoked have been published. Without the addition of blood biomarkers such as carcinoembryonic antigen (CEA), alpha-fetal protein, single nucleotide polymorphisms (SNPs) or spirometry data, the accuracy of these prediction models was modest (8,48-54). None of these models included ambient particulate matter pollution, the second most important cause of lung cancer deaths contributing to 15.1% of lung cancer deaths globally (55). Although the age-standardized death and incidence rates of lung cancer for both sexes combined decreased globally over the past decade, rates trended upwards for some populations, particularly females in certain countries lower on the socio-demographic index (SDI) (55). The age-standardized death rates of lung cancer attributable to ambient particulate matter pollution rose significantly in these populations over the past decade, while the age-standardized death rates attributable to household air pollution from solid fuels remained stagnant. Time-weighted mean exposure to particulate matter PM2.5 at the individual level since 1996 can be obtained from satellite observations, chemical transport models and ground measurements at high resolution (56). Research incorporating past air pollution exposure in lung cancer risk assessment to identify high-risk never smokers who may benefit from LDCT screening is urgently needed.
Equity in screening services
In the United States, screening uptake has been slow and varied widely across the country (57). Lung cancer screening rates were found not to be aligned with lung cancer burden across the US (57). Screening uptake was higher among individuals with insurance than among the uninsured (15.2% vs. 4.0%, P<0.001) (58). Uptake declined with age, higher education level, lack of primary care, lower provider knowledge of lung screening guideline and socioeconomic deprivation (59-61). Social deprivation is an independent predictor of lung cancer incidence and mortality (62). Using a social deprivation index constructed from community level education, income, unemployment, welfare assistance, and individual level covariates, the odds of lung cancer and death from lung cancer in the most deprived neighborhood were 1.27 and 1.32, respectively (62). Increasing travel time and level of urbanization have been associated with lower screening rates in established screening programs such as breast mammography and colorectal cancer screening (63-65). To facilitate planning for screening program growth and investment in the areas of greatest need, the American Cancer Society National Lung Cancer Round Table developed a geospatial mapping tool on the geographic distribution of tobacco use, incidence, and mortality of lung cancer, access to lung screening facilities, geographic disparities by race/ethnicity, income, health insurance, and rural/urban location in relation to travel distance (66,67). A similar geospatial mapping approach was used in the implementation of the British Columbia Lung Screening Program in Canada to map the location of the 36 screening sites. Utilizing lung cancer cases in health units across British Columbia as a proxy for the screen-eligible population, the impact of urbanization, individual components of the Statistics Canada’s Canadian Index of Multiple Deprivation, composed of sociodemographic and economic indicators, were considered in addition to vehicle travel time (68). Ninety-two percent of the lung cancer patients could access one of the 36 sites within one hour of driving. Longer drive times were found to be associated with situational vulnerability, economic dependency, residential instability, sex, and ethnocultural composition. The potential advantage of using lung cancer prevalence as a proxy for the screen-eligible population is that smoking history from electronic health records can be inaccurate and the smoking criteria for screening eligibility can change over time. The use of geospatial tools that take into account screening, diagnostic work-up and treatment resource capacity, disparity in access by Indigenous people, immigrants, social-economically deprived and underserved populations, to guide screening program improvement need to be evaluated. The potential application of machine learning tool using routine laboratory data such as complete blood count, in addition to clinical information to determine if a LDCT is warranted or the use of a point of care blood test as a pre-screening tool to optimize screening eligibility assessment (69,70) in underserved populations deserves further investigation.
Lung nodule management
An accurate, personalized screening LDCT management protocol is key to maximizing the benefits of screening in detecting lung cancer early while minimizing potential harms by reducing radiation exposure from unnecessary imaging studies, adverse events from biopsy or surgery for benign disease.
Most current lung cancer screening programs are based on a fixed, annual, screening interval until the upper age limit or until the participant would no longer benefit from screening, with shorter follow-up intervals to determine growth rate of suspicious nodules. Examples of these are the Lung Imaging Reporting and Data System (Lung-RADS) (71,72) and the EU-NELSON volumetric protocol (73). The International Lung Screening Trial (ILST) protocol is the only clinical protocol that has a biennial screening provision based on an individual’s lung cancer risk after the baseline (first) screening LDCT which comprise of approximately two-thirds of the screening population (74). The ILST protocol was based on the PanCan lung nodule malignancy risk calculator (75), that was reviewed by the Canadian Agency For Drugs And Technologies In Health comparing the diagnostic test accuracy of the PanCan versus Lung-RADS version 1.0 (76). In nine published studies, six indicate that the PanCan risk calculator performs better at determining which lung nodules identified by LDCT are cancerous compared to Lung-RADS with significantly better specificity and positive predictive value. PanCan had similar diagnostic test accuracy in three other studies—one case-control study, one study that only included subsolid nodules and the third study with a small sample size (76). A prospective study in Vancouver as part of the ILST showed a significantly higher sensitivity and positive predictive value of the PanCan protocol compared to Lung-RADS or the NELSON management protocols (77). A screening protocol with a biennial screening provision for lower risk individuals is currently used in the BC Lung Screening Program in British Columbia, Canada (78). This management protocol has significant implication in reducing health care resource utilization, cost and cumulative radiation exposure.
Other studies such as the randomized Multicentric Italian Lung Detection (MILD) trial compared annual vs. biennial LDCT screening interval and reported similar reduction in mortality with biennial screening which saved 44% of follow-up LDCT in subjects with negative baseline LDCT without increase in the occurrence of advanced lung cancer (79). A multicenter, multi-national trial, the 4-IN-THE-LUNG-RUN (4ITLR), is an on-going study in the EU to address the question if a 2 year instead of 1 year follow-up scan can be safely implemented in a screening program in participants with a negative baseline screen and lower lung cancer risk (80). Since findings in a prior scan (e.g., nodules versus no nodules, change in nodule size or attenuation), changes in smoking behavior and increasing age can modify lung cancer risk, several studies attempted to integrate this information to determine the timing of the next screening LDCT based on individual risk (33,81-85). Deep learning tools have also been developed to reduce the proportion of participants with indeterminate or intermediate risk lung nodules, personalize surveillance screening intervals and reduce radiologist workload (84,86-91). The risk thresholds to determine individually tailored screening schedules that optimize the benefits/harms trade-offs have not been prospectively evaluated in these studies. Whether one protocol is superior to another will require prospective randomized studies. Retrospective validation, while informative, is susceptible to bias due to the way individuals are followed based on recommendations from the standard of care rather than an artificial intelligence (AI) algorithm. For example, if AI recommends an earlier imaging study or biopsy for a more aggressive tumor, this benefit cannot be observed in retrospective data because the patient did not have a visit at this earlier time point, and it is unknown whether the patient already had lung cancer at that time.
The management of GGN is an area that requires further research; it has the greatest variation in management because of few high-quality studies on the natural history of these lesions (92). Lung-RADS version 1.1 increased the allowable nodule size for GGNs in category 2 from 20 to 30 mm. Chinese and Japanese guidelines, as well as the British Thoracic Society guideline, recommend early recall LDCT in 3–6 months for GGNs of at least 6 mm but less than 15 mm. Japanese and Chinese guidelines recommend diagnostic work-up for persistent nonsolid nodules of 15 mm or larger (93). The recommendation was based on the finding that a nodule larger than 15 mm is a significant determinant for invasive adenocarcinoma (94). A study using the NLST database suggested that if Lung-RADS categories for GGNs were based on malignancy probability similar to solid nodules, the GGN size threshold for early recall CT should be revised lower (6–19 mm) and not higher (95). In both Lung-RADS version 1.0 and version 1.1, no recommendation is made for biopsy until the nodule becomes part-solid or solid. Revising the size of GGNs from 20 to 30 mm showed no benefit but potentially missing some invasive adenocarcinoma (71).
Diagnostic work-up
Diagnostic work-up of a suspected lesion is an important part of the screening pathway as significant morbidity or even mortality can result from the diagnostic or surgical procedure. Balance must be made between over- and under-investigation of lesions, avoiding delays to diagnosis and treatment on one hand while minimizing harms of unnecessary procedures (5,6,96-98). Screen-detected early lung cancers create diagnostic challenges due to their small size and often peripheral location. In a screening trial, participants with a suspected early lung cancer on their CT scan who underwent surgical resection, the benign disease resection rate was as high as 34% (99). Pre-operative fine needle aspiration of the lung nodule can significantly reduce the nonmalignant surgical resection rate from 25.9% to 7.9% (100).
Positron emission tomography (PET) is recommended in several international guidelines for assessment of screen detected lung nodules (72,101,102). However, PET/CT has limited role for diagnosis of solid nodules in areas with endemic granulomatous diseases or for the diagnosis of non-solid nodules (103,104). Absent, or minimal increase in FDG uptake should not prevent biopsy or surgical resection of a sufficiently fast-growing nodule (93,103,105,106). The cost-effectiveness of routine PET/CT imaging for investigation of intermediate risk lung nodules versus volumetric growth measurement with repeat LDCT requires further investigation. The on-going Watch the Spot Trial may provide additional evidence to inform recommendations about management of patients with small lung nodules <15 mm (107).
PET/CT remains the mainstay of staging for lung cancer because the detection of unsuspected distant metastases or suspicious nodal disease on PET may profoundly influence selection of treatment. Patients with peripheral stage cIA1 tumors or ground glass opacities may not require a PET/CT for staging as nodal metastasis is known to be low in these lesions (108,109).
Tissue confirmation of malignancy in a small lung lesion can be challenging and requires an experienced operator. Options include bronchoscopic biopsy, CT-guided transthoracic biopsy or surgical resection. The choice of technique varies depending on nodule type and location, need for nodal sampling, patient comorbidities, operability and preference, as well as available local expertise and equipment. The overall diagnostic yield of navigational bronchoscopy with radial ultrasound-guided transbronchial lung biopsy showed an average diagnostic yield of 66% for lesions ≤20 mm and 81% for lesions between 20 to 30 mm compared to 92% and 96% respectively with CT-guided transthoracic biopsy (110). Bronchoscopic biopsies had significantly lower rates of pneumothorax and bleeding (93,110,111). ‘Real-world’ experience with CT-guided biopsies of small screen-detected lesions can be lower (78%) (112).
Since the major downsides of screening are complications related to radiation exposure, biopsies or surgery for benign disease, best practices need to be evaluated using quality indicators such as percent of LDCT screens which recommended additional imaging studies, positive predictive values of a diagnostic work-up biopsy and resection rate of benign lesions (113).
Additional findings on screening LDCT
Additional findings (incidental findings) are commonly encountered in a screening LDCT. These findings are unrelated to the goal of the study which is for lung cancer screening, Examples of these are moderate or greater coronary artery calcification (CAC), moderate or severe emphysema, interstitial lung disease including pulmonary fibrosis, a mass in the neck, chest or upper abdomen, aortic aneurysms, bronchiectasis or osteoporosis (114-116). Only findings that have clinical implication should be reported as investigations may add considerable cost to screening program and cause harm to the patient (117). When reported, information should be provided to the health care provider regarding the recommended next step.
Smoking cessation
By design, lung cancer screening programs attract participants with smoking rates above the community average. In the 50 to 80 years age group, about 50% of screening participants are still smoking (5,6,18,19,34,118-121) compared to 10% to 15% in the general population. In the Italian COSMOS study, a significantly higher incidence of lung cancer was observed in those who continued to smoke following the first screening LDCT (122). In the NLST, 7 years of abstinence from tobacco smoking alone was associated with 20% reduction in lung cancer mortality (123). Combining smoking abstinence with LDCT screening resulted in 38% reduction in death from Lung cancer (123). A 39% reduction in all-cause mortality was observed in former compared to current smokers undergoing repeated LDCT screening in the MILD trial (124). One-third of the current smokers in NLST were found to be highly dependent on tobacco (125). High tobacco dependency was associated with higher lung cancer rates, all-cause mortality and lung cancer-specific mortality (125). A recent lung cancer risk modeling study that integrates past screening findings and changes in smoking behavior of individuals suggested people who continued to smoke heavily would require annual screening above the age of 61 even with two consecutive negative screens while those who stopped smoking could have biennial screening (126). Since smoking cessation prevents the development of lung cancer, reduces lung cancer specific and all-cause mortality and is cost-effective (127-129), it is imperative that smoking cessation programs are integrated in lung cancer screening programs (130).
The optimal interventions to facilitate smoking cessation in the screening setting is the subject of ongoing clinical trials (131,132). The average quit rate with counseling is between 11% to 14% (133). The cessation rates are higher with more than one screening round and longer duration in the screening program (134,135). Abnormal findings on a CT scan may increase initial quit attempts especially if the finding is new and suspicious for lung cancer but the behavior for less significant abnormality may not be sustained (133,136,137). A randomized telephone-based smoking cessation counseling intervention incorporating lung cancer screening results did not result in increased 12-month cessation rates versus written information alone in unselected smokers undergoing lung cancer screening (138). In another randomized trial, more intensive telephone counseling comparing eight 20 minutes phone sessions + 8 weeks nicotine patch was compared with minimal three 20 minutes phone sessions + 2 weeks of optional nicotine patch. A higher quit rate was observed with the intensive intervention only in the first 3 months which was probably related to the addition of nicotine replacement therapy (139).
A significant proportion of the current smokers in screening studies such as the NLST were found to be highly dependent on tobacco with 34% reported time to first cigarette of less than 5 minutes (125). The likelihood of cessation decreased with incremental increases in severity of dependence. Studies have shown combining counseling with pharmacotherapy can double the quit rate and that those with high nicotine dependence benefitted most from dual pharmacologic therapy (140-142). In the NLST, only a minority of smoking participants were offered smoking cessation pharmacotherapy (142). In a Veterans Health Administration study, only 1.1% received the recommended combination of pharmacotherapy and counseling; of those receiving pharmacotherapy, only one in four received one of the most effective medications: varenicline (12.1%) or combination nicotine replacement therapy (14.3%) (143). Cost and the need for a prescription may be the issue. Cytisine, an inexpensive partial agonist binding nicotine acetylcholine receptor nicotinic receptor similar to varenicline may be an option—achieving a 32% 12-month quit rate compared to 7% with counselling alone (144). Treatment of tobacco dependency and evaluation of the success of smoking cessation programs should consider the level of nicotine dependency which can be readily assessed by asking a simple question on time to first cigarette upon waking (125). Engagement of primary care providers to provide pharmacotherapy needs to be improved. Lobbying governments to provide free nicotine replacement therapy and other smoking cessation drugs should be a priority.
Individuals who are still smoking but are ineligible for lung cancer screening should be offered smoking cessation. Only a minority of this group was found to participate in smoking cessation programs but almost all were interested in quitting and in receiving help with quit attempts (145).
Conclusions
LDCT screening for lung cancer significantly reduces mortality from lung cancer. To maximize the benefits of LDCT screening, research is needed to identify high risk individuals who are not currently eligible but would benefit from LDCT screening. The role of blood or breath biomarkers should be evaluated for potential application to identify high risk individuals for LDCT screening (146). AI and deep learning methods integrating clinical, demographic and imaging information are promising approaches to personalize the screening interval and duration should be evaluated in randomized controlled studies. Future studies are needed to determine the most effective approaches to deliver smoking cessation services to large numbers of older individuals who have been smoking for several decades and are still smoking. LDCT screening goes beyond detecting and treating lung cancer early; it provides the framework for improving lung cancer care in the general population through prevention, risk assessment, early detection, rapid diagnosis and timely treatment.
Acknowledgments
Funding: None.
Footnote
Provenance and Peer Review: This article was commissioned by the Guest Editors (Donna E. Maziak and Patrick J. Villeneuve) for the series “Comprehensive Lung Cancer Care: A Continuum” published in Current Challenges in Thoracic Surgery. The article has undergone external peer review.
Reporting Checklist: The authors have completed the Narrative Review reporting checklist. Available at https://ccts.amegroups.com/article/view/10.21037/ccts-23-3/rc
Peer Review File: Available at https://ccts.amegroups.com/article/view/10.21037/ccts-23-3/prf
Conflicts of Interest: Both authors have completed the ICMJE uniform disclosure form (available at https://ccts.amegroups.com/article/view/10.21037/ccts-23-3/coif). The series “Comprehensive Lung Cancer Care: A Continuum” was commissioned by the editorial office without any funding or sponsorship. SL received research grants from the Terry Fox Research Institute and the National Institute of Health (USA). He receives consultant fee as an expert advisor for the Canadian Partnership Against Cancer. He is a member of the Medical Advisory Board of Nucelix Inc. (unpaid). He has partial travel support from the International Association for the Study of Lung Cancer to participate in the World Conference on Lung Cancer. The authors have no other conflicts of interest to declare.
Ethical Statement: The authors are accountable for all aspects of the work in ensuring that questions related to the accuracy or integrity of any part of the work are appropriately investigated and resolved.
Open Access Statement: This is an Open Access article distributed in accordance with the Creative Commons Attribution-NonCommercial-NoDerivs 4.0 International License (CC BY-NC-ND 4.0), which permits the non-commercial replication and distribution of the article with the strict proviso that no changes or edits are made and the original work is properly cited (including links to both the formal publication through the relevant DOI and the license). See: https://creativecommons.org/licenses/by-nc-nd/4.0/.
References
- Sung H, Ferlay J, Siegel RL, et al. Global Cancer Statistics 2020: GLOBOCAN Estimates of Incidence and Mortality Worldwide for 36 Cancers in 185 Countries. CA Cancer J Clin 2021;71:209-49. [Crossref] [PubMed]
- Bonney A, Malouf R, Marchal C, et al. Impact of low-dose computed tomography (LDCT) screening on lung cancer-related mortality. Cochrane Database Syst Rev 2022;8:CD013829. [PubMed]
- Field JK, Vulkan D, Davies MPA, et al. Lung cancer mortality reduction by LDCT screening: UKLS randomised trial results and international meta-analysis. Lancet Reg Health Eur 2021;10:100179. [Crossref] [PubMed]
- Passiglia F, Cinquini M, Bertolaccini L, et al. Benefits and Harms of Lung Cancer Screening by Chest Computed Tomography: A Systematic Review and Meta-Analysis. J Clin Oncol 2021;39:2574-85. [Crossref] [PubMed]
- National Lung Screening Trial Research Team. Reduced lung-cancer mortality with low-dose computed tomographic screening. N Engl J Med 2011;365:395-409. [Crossref] [PubMed]
- de Koning HJ, van der Aalst CM, de Jong PA, et al. Reduced Lung-Cancer Mortality with Volume CT Screening in a Randomized Trial. N Engl J Med 2020;382:503-13. [Crossref] [PubMed]
- Network LCP [Internet]. Interactive map of lung cancer screening 2022. [cited 2023 April 28]. Available online: https://www.lungcancerpolicynetwork.com/interactive-map-of-lung-cancer-screening/
- Tammemägi MC, Church TR, Hocking WG, et al. Evaluation of the lung cancer risks at which to screen ever- and never-smokers: screening rules applied to the PLCO and NLST cohorts. PLoS Med 2014;11:e1001764. [Crossref] [PubMed]
- Moyer VA. U.S. Screening for lung cancer: U.S. Preventive Services Task Force recommendation statement. Ann Intern Med 2014;160:330-8. [Crossref] [PubMed]
- Centers for Medicare & Medicaid Services (CMS) [Internet]. Decision Memo for Screening for Lung Cancer with Low Dose Computed Tomography (LDCT) (CAG-00439N). [cited 2023 April 28]. Available online: https://www.cms.gov/medicare-coverage-database/details/nca-decision-memo.aspx?NCAId=274&NcaName=Screening+for+Lung+Cancer+with+Low+Dose+Computed+Tomography+(LDCT)&MEDCACId=68&IsPopup=y&bc=AAAAAAAAAgAAAA%3d%3d&
- US Preventive Services Task Force. Screening for Lung Cancer: US Preventive Services Task Force Recommendation Statement. JAMA 2021;325:962-70. [Crossref] [PubMed]
- Recommendations on screening for lung cancer. CMAJ 2016;188:425-32. [Crossref] [PubMed]
- Tammemägi MC, Katki HA, Hocking WG, et al. Selection criteria for lung-cancer screening. N Engl J Med 2013;368:728-36. [Crossref] [PubMed]
- Tammemägi MC. Application of risk prediction models to lung cancer screening: a review. J Thorac Imaging 2015;30:88-100. [Crossref] [PubMed]
- Bach PB, Kattan MW, Thornquist MD, et al. Variations in lung cancer risk among smokers. J Natl Cancer Inst 2003;95:470-8. [Crossref] [PubMed]
- Katki HA, Kovalchik SA, Berg CD, et al. Development and Validation of Risk Models to Select Ever-Smokers for CT Lung Cancer Screening. JAMA 2016;315:2300-11. [Crossref] [PubMed]
- Marcus MW, Chen Y, Raji OY, et al. LLPi: Liverpool Lung Project Risk Prediction Model for Lung Cancer Incidence. Cancer Prev Res (Phila) 2015;8:570-5. [Crossref] [PubMed]
- Tammemagi MC, Schmidt H, Martel S, et al. Participant selection for lung cancer screening by risk modelling (the Pan-Canadian Early Detection of Lung Cancer [PanCan] study): a single-arm, prospective study. Lancet Oncol 2017;18:1523-31. [Crossref] [PubMed]
- Crosbie PA, Balata H, Evison M, et al. Implementing lung cancer screening: baseline results from a community-based 'Lung Health Check' pilot in deprived areas of Manchester. Thorax 2019;74:405-9. [Crossref] [PubMed]
- Tammemägi MC. Selecting lung cancer screenees using risk prediction models-where do we go from here. Transl Lung Cancer Res 2018;7:243-53. [Crossref] [PubMed]
- Ten Haaf K, Bastani M, Cao P, et al. A Comparative Modeling Analysis of Risk-Based Lung Cancer Screening Strategies. J Natl Cancer Inst 2020;112:466-79. [Crossref] [PubMed]
- Katki HA, Kovalchik SA, Petito LC, et al. Implications of Nine Risk Prediction Models for Selecting Ever-Smokers for Computed Tomography Lung Cancer Screening. Ann Intern Med 2018;169:10-9. [Crossref] [PubMed]
- Li K, Hüsing A, Sookthai D, et al. Selecting High-Risk Individuals for Lung Cancer Screening: A Prospective Evaluation of Existing Risk Models and Eligibility Criteria in the German EPIC Cohort. Cancer Prev Res (Phila) 2015;8:777-85. [Crossref] [PubMed]
- Hüsing A, Kaaks R. Risk prediction models versus simplified selection criteria to determine eligibility for lung cancer screening: an analysis of German federal-wide survey and incidence data. Eur J Epidemiol 2020;35:899-912. [Crossref] [PubMed]
- Weber M, Yap S, Goldsbury D, et al. Identifying high risk individuals for targeted lung cancer screening: Independent validation of the PLCO(m2012) risk prediction tool. Int J Cancer 2017;141:242-53. [Crossref] [PubMed]
- Ten Haaf K, Jeon J, Tammemägi MC, et al. Risk prediction models for selection of lung cancer screening candidates: A retrospective validation study. PLoS Med 2017;14:e1002277. [Crossref] [PubMed]
- Kavanagh J, Liu G, Menezes R, et al. Importance of Long-term Low-Dose CT Follow-up after Negative Findings at Previous Lung Cancer Screening. Radiology 2018;289:218-24. [Crossref] [PubMed]
- Aggarwal R, Lam ACL, McGregor M, et al. Outcomes of Long-term Interval Rescreening With Low-Dose Computed Tomography for Lung Cancer in Different Risk Cohorts. J Thorac Oncol 2019;14:1003-11. [Crossref] [PubMed]
- Meza R, Jeon J, Toumazis I, et al. Evaluation of the Benefits and Harms of Lung Cancer Screening With Low-Dose Computed Tomography: Modeling Study for the US Preventive Services Task Force. JAMA 2021;325:988-97. [Crossref] [PubMed]
- Cressman S, Peacock SJ, Tammemägi MC, et al. The Cost-Effectiveness of High-Risk Lung Cancer Screening and Drivers of Program Efficiency. J Thorac Oncol 2017;12:1210-22. [Crossref] [PubMed]
- Grover H, King W, Bhattarai N, et al. Systematic review of the cost-effectiveness of screening for lung cancer with low dose computed tomography. Lung Cancer 2022;170:20-33. [Crossref] [PubMed]
- Cressman S, Weber MF, Ngo PJ, et al. Economic impact of using risk models for eligibility selection to the International lung screening Trial. Lung Cancer 2023;176:38-45. [Crossref] [PubMed]
- Toumazis I, Cao P, de Nijs K, et al. Risk Model-Based Lung Cancer Screening: A Cost-Effectiveness Analysis. Ann Intern Med 2023;176:320-32. [Crossref] [PubMed]
- Tammemägi MC, Ruparel M, Tremblay A, et al. USPSTF2013 versus PLCOm2012 lung cancer screening eligibility criteria (International Lung Screening Trial): interim analysis of a prospective cohort study. Lancet Oncol 2022;23:138-48. [Crossref] [PubMed]
- Pasquinelli MM, Tammemägi MC, Kovitz KL, et al. Addressing Sex Disparities in Lung Cancer Screening Eligibility: USPSTF vs PLCOm2012 Criteria. Chest 2022;161:248-56. [Crossref] [PubMed]
- Gionet L, Roshanafshar S. Select health indicators of First Nations people living off reserve, Métis and Inuit. [Internet]. Statistics Canada; 2013 [cited 2023 April 28]. Available online: https://www150.statcan.gc.ca/n1/pub/82-624-x/2013001/article/11763-eng.htm
- Pasquinelli MM, Tammemägi MC, Kovitz KL, et al. Brief Report: Risk Prediction Model Versus United States Preventive Services Task Force 2020 Draft Lung Cancer Screening Eligibility Criteria-Reducing Race Disparities. JTO Clin Res Rep 2020;2:100137. [PubMed]
- Reitsma M, Kendrick P, Anderson J, et al. Reexamining Rates of Decline in Lung Cancer Risk after Smoking Cessation. A Meta-analysis. Ann Am Thorac Soc 2020;17:1126-32. [Crossref] [PubMed]
- Canadian Cancer Statistics Advisory Committee. Canadian cancer statistics. A 2020 special report on lung cancer. [Internet]. Canadian Cancer Society. [cited 2023 April 28]. Available online: www.cancer.ca/Canadian-Cancer-Statistics-2020-EN
- Huber RM, Cavic M, Kerpel-Fronius A, et al. Lung Cancer Screening Considerations During Respiratory Infection Outbreaks, Epidemics or Pandemics: An International Association for the Study of Lung Cancer Early Detection and Screening Committee Report. J Thorac Oncol 2022;17:228-38. [Crossref] [PubMed]
- Chien LH, Chen TY, Chen CH, et al. Recalibrating Risk Prediction Models by Synthesizing Data Sources: Adapting the Lung Cancer PLCO Model for Taiwan. Cancer Epidemiol Biomarkers Prev 2022;31:2208-18. [Crossref] [PubMed]
- Jeon J, Holford TR, Levy DT, et al. Smoking and Lung Cancer Mortality in the United States From 2015 to 2065: A Comparative Modeling Approach. Ann Intern Med 2018;169:684-93. [Crossref] [PubMed]
- Tseng CH, Tsuang BJ, Chiang CJ, et al. The Relationship Between Air Pollution and Lung Cancer in Nonsmokers in Taiwan. J Thorac Oncol 2019;14:784-92. [Crossref] [PubMed]
- Yang P. PS01.02 National Lung Cancer Screening Program in Taiwan: The TALENT Study. J Thorac Oncol 2021;16:S58. [Crossref]
- Nawa T, Nakagawa T, Mizoue T, et al. A decrease in lung cancer mortality following the introduction of low-dose chest CT screening in Hitachi, Japan. Lung Cancer 2012;78:225-8. [Crossref] [PubMed]
- Gao W, Wen CP, Wu A, et al. Association of Computed Tomographic Screening Promotion With Lung Cancer Overdiagnosis Among Asian Women. JAMA Intern Med 2022;182:283-90. [Crossref] [PubMed]
- Wang M, Lin S, He N, et al. The Introduction of Low-Dose CT Imaging and Lung Cancer Overdiagnosis in Chinese Women. Chest 2023;163:239-50. [Crossref] [PubMed]
- Lam S. Lung Cancer Screening in Never-Smokers. J Thorac Oncol 2019;14:336-7. [Crossref] [PubMed]
- Chien LH, Chen CH, Chen TY, et al. Predicting Lung Cancer Occurrence in Never-Smoking Females in Asia: TNSF-SQ, a Prediction Model. Cancer Epidemiol Biomarkers Prev 2020;29:452-9. [Crossref] [PubMed]
- Warkentin MT, Lam S, Hung RJ. Determinants of impaired lung function and lung cancer prediction among never-smokers in the UK Biobank cohort. EBioMedicine 2019;47:58-64. [Crossref] [PubMed]
- Wu X, Wen CP, Ye Y, et al. Personalized Risk Assessment in Never, Light, and Heavy Smokers in a prospective cohort in Taiwan. Sci Rep 2016;6:36482. [Crossref] [PubMed]
- Wu X, Wen CP, Ye Y, et al. Author Correction: Personalized Risk Assessment in Never, Light, and Heavy Smokers in a prospective cohort in Taiwan. Sci Rep 2020;10:5514. [Crossref] [PubMed]
- Wang F, Tan F, Shen S, et al. Risk-stratified Approach for Never- and Ever-Smokers in Lung Cancer Screening: A Prospective Cohort Study in China. Am J Respir Crit Care Med 2023;207:77-88. [Crossref] [PubMed]
- Bates JHT, Hamlington KL, Garrison G, et al. Prediction of lung cancer risk based on age and smoking history. Comput Methods Programs Biomed 2022;216:106660. [Crossref] [PubMed]
- Global, regional, and national burden of respiratory tract cancers and associated risk factors from 1990 to 2019: a systematic analysis for the Global Burden of Disease Study 2019. Lancet Respir Med 2021;9:1030-49. [Crossref] [PubMed]
- Myers R, Brauer M, Dummer T, et al. High-Ambient Air Pollution Exposure Among Never Smokers Versus Ever Smokers With Lung Cancer. J Thorac Oncol 2021;16:1850-8. [Crossref] [PubMed]
- Fedewa SA, Kazerooni EA, Studts JL, et al. State Variation in Low-Dose Computed Tomography Scanning for Lung Cancer Screening in the United States. J Natl Cancer Inst 2021;113:1044-52. [Crossref] [PubMed]
- Zahnd WE, Eberth JM. Lung Cancer Screening Utilization: A Behavioral Risk Factor Surveillance System Analysis. Am J Prev Med 2019;57:250-5. [Crossref] [PubMed]
- Zgodic A, Zahnd WE, Advani S, et al. Low-dose CT lung cancer screening uptake: A rural-urban comparison. J Rural Health 2022;38:40-53. [Crossref] [PubMed]
- Quaife SL, Ruparel M, Dickson JL, et al. Lung Screen Uptake Trial (LSUT): Randomized Controlled Clinical Trial Testing Targeted Invitation Materials. Am J Respir Crit Care Med 2020;201:965-75. [Crossref] [PubMed]
- Lewis JA, Chen H, Weaver KE, et al. Low Provider Knowledge Is Associated With Less Evidence-Based Lung Cancer Screening. J Natl Compr Canc Netw 2019;17:339-46. [Crossref] [PubMed]
- Li X, Sundquist J, Zöller B, et al. Neighborhood deprivation and lung cancer incidence and mortality: a multilevel analysis from Sweden. J Thorac Oncol 2015;10:256-63. [Crossref] [PubMed]
- Simkin J, Ogilvie G, Hanley B, et al. Differences in colorectal cancer screening rates across income strata by levels of urbanization: results from the Canadian Community Health Survey (2013/2014) Can J Public Health 2019;110:62-71. [Crossref] [PubMed]
- St-Jacques S, Philibert MD, Langlois A, et al. Geographic access to mammography screening centre and participation of women in the Quebec Breast Cancer Screening Programme. J Epidemiol Community Health 2013;67:861-7. [Crossref] [PubMed]
- Kerner J, Liu J, Wang K, et al. Canadian cancer screening disparities: a recent historical perspective. Curr Oncol 2015;22:156-63. [Crossref] [PubMed]
- Sahar L, Douangchai Wills VL, Liu KK, et al. Using Geospatial Analysis to Evaluate Access to Lung Cancer Screening in the United States. Chest 2021;159:833-44. [Crossref] [PubMed]
- Sahar L, Douangchai Wills VL, Liu KKA, et al. Geographic access to lung cancer screening among eligible adults living in rural and urban environments in the United States. Cancer 2022;128:1584-94. [Crossref] [PubMed]
- Simkin J, Khoo E, Darvishian M, et al. Addressing Inequity in Spatial Access to Lung Cancer Screening. Curr Oncol 2023;30:8078-91. [Crossref] [PubMed]
- Gould MK, Huang BZ, Tammemagi MC, et al. Machine Learning for Early Lung Cancer Identification Using Routine Clinical and Laboratory Data. Am J Respir Crit Care Med 2021;204:445-53. [Crossref] [PubMed]
- Fahrmann JF, Marsh T, Irajizad E, et al. Blood-Based Biomarker Panel for Personalized Lung Cancer Risk Assessment. J Clin Oncol 2022;40:876-83. [Crossref] [PubMed]
- Kastner J, Hossain R, Jeudy J, et al. Lung-RADS Version 1.0 versus Lung-RADS Version 1.1: Comparison of Categories Using Nodules from the National Lung Screening Trial. Radiology 2021;300:199-206. [Crossref] [PubMed]
- Chelala L, Hossain R, Kazerooni EA, et al. Lung-RADS Version 1.1: Challenges and a Look Ahead, From the AJR Special Series on Radiology Reporting and Data Systems. AJR Am J Roentgenol 2021;216:1411-22. [Crossref] [PubMed]
- Oudkerk M, Devaraj A, Vliegenthart R, et al. European position statement on lung cancer screening. Lancet Oncol 2017;18:e754-66. [Crossref] [PubMed]
- Lim KP, Marshall H, Tammemägi M, et al. Protocol and Rationale for the International Lung Screening Trial. Ann Am Thorac Soc 2020;17:503-12. [Crossref] [PubMed]
- McWilliams A, Tammemagi MC, Mayo JR, et al. Probability of cancer in pulmonary nodules detected on first screening CT. N Engl J Med 2013;369:910-9. [Crossref] [PubMed]
- Barbara AM, Loshak H. Lung-RADS Versus Pan-Canadian Early Detection of Lung Cancer Study Screening for Patients at High Risk of Lung Cancer. Can J Health Technol 2021; [Crossref]
- Myers R, Mayo J, Atkar-Khattra S, et al. MA10.01 Prospective Evaluation of the International Lung Screening Trial (ILST) Protocol for Management of First Screening LDCT. J Thorac Oncol 2021;16:S913-4. [Crossref]
- BC Cancer [Internet]. Lung Screening Standards and Protocols. [cited 2023 April 28]. Available online: http://www.bccancer.bc.ca/screening/Documents/Lung-Screening-Standards-Protocols.pdf
- Pastorino U, Silva M, Sestini S, et al. Prolonged lung cancer screening reduced 10-year mortality in the MILD trial: new confirmation of lung cancer screening efficacy. Ann Oncol 2019;30:1162-9. [Crossref] [PubMed]
- CORDIS. EU research results. [Internet]. 4-IN THE LUNG RUN: towards INdividually tailored INvitations, screening INtervals, and INtegrated co-morbidity reducing strategies in lung cancer screening. 2022. [cited 2023 April 28] Available online: https://cordis.europa.eu/project/id/848294
- Tammemägi MC, Ten Haaf K, Toumazis I, et al. Development and Validation of a Multivariable Lung Cancer Risk Prediction Model That Includes Low-Dose Computed Tomography Screening Results: A Secondary Analysis of Data From the National Lung Screening Trial. JAMA Netw Open 2019;2:e190204. [Crossref] [PubMed]
- Robbins HA, Cheung LC, Chaturvedi AK, et al. Management of Lung Cancer Screening Results Based on Individual Prediction of Current and Future Lung Cancer Risks. J Thorac Oncol 2022;17:252-63. [Crossref] [PubMed]
- Robbins HA, Berg CD, Cheung LC, et al. Identification of Candidates for Longer Lung Cancer Screening Intervals Following a Negative Low-Dose Computed Tomography Result. J Natl Cancer Inst 2019;111:996-9. [Crossref] [PubMed]
- Huang P, Lin CT, Li Y, et al. Prediction of lung cancer risk at follow-up screening with low-dose CT: a training and validation study of a deep learning method. Lancet Digit Health 2019;1:e353-62. [Crossref] [PubMed]
- Cao P, Jeon J, Meza R. Evaluation of benefits and harms of adaptive screening schedules for lung cancer: A microsimulation study. J Med Screen 2022;29:260-7. [Crossref] [PubMed]
- Lancaster HL, Zheng S, Aleshina OO, et al. Outstanding negative prediction performance of solid pulmonary nodule volume AI for ultra-LDCT baseline lung cancer screening risk stratification. Lung Cancer 2022;165:133-40. [Crossref] [PubMed]
- Baldwin DR, Gustafson J, Pickup L, et al. External validation of a convolutional neural network artificial intelligence tool to predict malignancy in pulmonary nodules. Thorax 2020;75:306-12. [Crossref] [PubMed]
- Heuvelmans MA, van Ooijen PMA, Ather S, et al. Lung cancer prediction by Deep Learning to identify benign lung nodules. Lung Cancer 2021;154:1-4. [Crossref] [PubMed]
- Massion PP, Antic S, Ather S, et al. Assessing the Accuracy of a Deep Learning Method to Risk Stratify Indeterminate Pulmonary Nodules. Am J Respir Crit Care Med 2020;202:241-9. [Crossref] [PubMed]
- Schreuder A, Scholten ET, van Ginneken B, et al. Artificial intelligence for detection and characterization of pulmonary nodules in lung cancer CT screening: ready for practice? Transl Lung Cancer Res 2021;10:2378-88. [Crossref] [PubMed]
- Mikhael PG, Wohlwend J, Yala A, et al. Sybil: A Validated Deep Learning Model to Predict Future Lung Cancer Risk From a Single Low-Dose Chest Computed Tomography. J Clin Oncol 2023;41:2191-200. [Crossref] [PubMed]
- Mayo JR, Lam S. Doing Too Much or Not Enough: Striking a Balance. Radiology 2021;300:207-8. [Crossref] [PubMed]
- Lam S, Bryant H, Donahoe L, et al. Management of screen-detected lung nodules: A Canadian partnership against cancer guidance document. Canadian Journal of Respiratory, Critical Care, and Sleep Medicine 2020;4:236-65. [Crossref]
- Chang B, Hwang JH, Choi YH, et al. Natural history of pure ground-glass opacity lung nodules detected by low-dose CT scan. Chest 2013;143:172-8. [Crossref] [PubMed]
- Robbins HA, Katki HA, Cheung LC, et al. Insights for Management of Ground-Glass Opacities From the National Lung Screening Trial. J Thorac Oncol 2019;14:1662-5. [Crossref] [PubMed]
- Flores R, Bauer T, Aye R, et al. Balancing curability and unnecessary surgery in the context of computed tomography screening for lung cancer. J Thorac Cardiovasc Surg 2014;147:1619-26. [Crossref] [PubMed]
- Van't Westeinde SC, Horeweg N, De Leyn P, et al. Complications following lung surgery in the Dutch-Belgian randomized lung cancer screening trial. Eur J Cardiothorac Surg 2012;42:420-9. [Crossref] [PubMed]
- Yankelevitz DF, Yip R, Henschke CI. Impact of Duration of Diagnostic Workup on Prognosis for Early Lung Cancer. J Thorac Oncol 2023;18:527-37. [Crossref] [PubMed]
- Wilson DO, Weissfeld JL, Fuhrman CR, et al. The Pittsburgh Lung Screening Study (PLuSS): outcomes within 3 years of a first computed tomography scan. Am J Respir Crit Care Med 2008;178:956-61. [Crossref] [PubMed]
- Barta JA, Henschke CI, Flores RM, et al. Lung Cancer Diagnosis by Fine Needle Aspiration Is Associated With Reduction in Resection of Nonmalignant Lung Nodules. Ann Thorac Surg 2017;103:1795-801. [Crossref] [PubMed]
- Callister ME, Baldwin DR, Akram AR, et al. British Thoracic Society guidelines for the investigation and management of pulmonary nodules. Thorax 2015;70:ii1-ii54. [Crossref] [PubMed]
- Wood DE, Kazerooni EA, Baum SL, et al. Lung Cancer Screening, Version 3.2018, NCCN Clinical Practice Guidelines in Oncology. J Natl Compr Canc Netw 2018;16:412-41. [Crossref] [PubMed]
- Maiga AW, Deppen SA, Mercaldo SF, et al. Assessment of Fluorodeoxyglucose F18-Labeled Positron Emission Tomography for Diagnosis of High-Risk Lung Nodules. JAMA Surg 2018;153:329-34. [Crossref] [PubMed]
- Deppen SA, Blume JD, Kensinger CD, et al. Accuracy of FDG-PET to diagnose lung cancer in areas with infectious lung disease: a meta-analysis. JAMA 2014;312:1227-36. [Crossref] [PubMed]
- van't Westeinde SC, de Koning HJ, Thunnissen FB, et al. The role of the 18f-fluorodeoxyglucose-positron emission tomography scan in the Nederlands Leuvens Longkanker screenings Onderzoek lung cancer screening trial. J Thorac Oncol 2011;6:1704-12. [Crossref] [PubMed]
- Veronesi G, Travaini LL, Maisonneuve P, et al. Positron emission tomography in the diagnostic work-up of screening-detected lung nodules. Eur Respir J 2015;45:501-10. [Crossref] [PubMed]
- Gould MK, Smith-Bindman R, Kelly K, et al. Methods for the Watch the Spot Trial. A Pragmatic Trial of More- versus Less-Intensive Strategies for Active Surveillance of Small Pulmonary Nodules. Ann Am Thorac Soc 2019;16:1567-76. [Crossref] [PubMed]
- Ye B, Cheng M, Li W, et al. Predictive factors for lymph node metastasis in clinical stage IA lung adenocarcinoma. Ann Thorac Surg 2014;98:217-23. [Crossref] [PubMed]
- Cho S, Song IH, Yang HC, et al. Predictive factors for node metastasis in patients with clinical stage I non-small cell lung cancer. Ann Thorac Surg 2013;96:239-45. [Crossref] [PubMed]
- Han Y, Kim HJ, Kong KA, et al. Diagnosis of small pulmonary lesions by transbronchial lung biopsy with radial endobronchial ultrasound and virtual bronchoscopic navigation versus CT-guided transthoracic needle biopsy: A systematic review and meta-analysis. PLoS One 2018;13:e0191590. [Crossref] [PubMed]
- McGuire AL, Myers R, Grant K, et al. The Diagnostic Accuracy and Sensitivity for Malignancy of Radial-Endobronchial Ultrasound and Electromagnetic Navigation Bronchoscopy for Sampling of Peripheral Pulmonary Lesions: Systematic Review and Meta-analysis. J Bronchology Interv Pulmonol 2020;27:106-21. [Crossref] [PubMed]
- Wagnetz U, Menezes RJ, Boerner S, et al. CT screening for lung cancer: implication of lung biopsy recommendations. AJR Am J Roentgenol 2012;198:351-8. [Crossref] [PubMed]
- Selby K, Sedki M, Levine E, et al. Test performance metrics for breast, cervical, colon, and lung cancer screening: a systematic review. J Natl Cancer Inst 2023;115:375-84. [Crossref] [PubMed]
- Radiology ACR [Internet]. ACR Lung Cancer Screening CT Incidental Findings. [cited 2023 April 28]. Available online: https://www.acraccreditation.org/-/media/ACRAccreditation/Documents/LCS/LCS-Incidental-Findings-Quick-Guide-Oct-2021.pdf
- Tanoue LT, Sather P, Cortopassi I, et al. Standardizing the Reporting of Incidental, Non-Lung Cancer (Category S) Findings Identified on Lung Cancer Screening Low-Dose CT Imaging. Chest 2022;161:1697-706. [Crossref] [PubMed]
- Dyer DS, White C, Conley Thomson C, et al. A Quick Reference Guide for Incidental Findings on Lung Cancer Screening CT Examinations. J Am Coll Radiol 2023;20:162-72. [Crossref] [PubMed]
- van de Wiel JC, Wang Y, Xu DM, et al. Neglectable benefit of searching for incidental findings in the Dutch-Belgian lung cancer screening trial (NELSON) using low-dose multidetector CT. Eur Radiol 2007;17:1474-82. [Crossref] [PubMed]
- Becker N, Motsch E, Trotter A, et al. Lung cancer mortality reduction by LDCT screening-Results from the randomized German LUSI trial. Int J Cancer 2020;146:1503-13. [Crossref] [PubMed]
- Field JK, Duffy SW, Baldwin DR, et al. The UK Lung Cancer Screening Trial: a pilot randomised controlled trial of low-dose computed tomography screening for the early detection of lung cancer. Health Technol Assess 2016;20:1-146. [Crossref] [PubMed]
- Richards TB, Soman A, Thomas CC, et al. Screening for Lung Cancer - 10 States, 2017. MMWR Morb Mortal Wkly Rep 2020;69:201-6. [Crossref] [PubMed]
- Foxon F, Selya A, Gitchell J, et al. Population-level counterfactual trend modelling to examine the relationship between smoking prevalence and e-cigarette use among US adults. BMC Public Health 2022;22:1940. [Crossref] [PubMed]
- Veronesi G, Maisonneuve P, Rampinelli C, et al. Computed tomography screening for lung cancer: results of ten years of annual screening and validation of cosmos prediction model. Lung Cancer 2013;82:426-30. [Crossref] [PubMed]
- Tanner NT, Kanodra NM, Gebregziabher M, et al. The Association between Smoking Abstinence and Mortality in the National Lung Screening Trial. Am J Respir Crit Care Med 2016;193:534-41. [Crossref] [PubMed]
- Pastorino U, Boffi R, Marchianò A, et al. Stopping Smoking Reduces Mortality in Low-Dose Computed Tomography Screening Participants. J Thorac Oncol 2016;11:693-9. [Crossref] [PubMed]
- Rojewski AM, Tanner NT, Dai L, et al. Tobacco Dependence Predicts Higher Lung Cancer and Mortality Rates and Lower Rates of Smoking Cessation in the National Lung Screening Trial. Chest 2018;154:110-8. [Crossref] [PubMed]
- Toumazis I, Alagoz O, Leung A, et al. A risk-based framework for assessing real-time lung cancer screening eligibility that incorporates life expectancy and past screening findings. Cancer 2021;127:4432-46. [Crossref] [PubMed]
- Evans WK, Gauvreau CL, Flanagan WM, et al. Clinical impact and cost-effectiveness of integrating smoking cessation into lung cancer screening: a microsimulation model. CMAJ Open 2020;8:E585-92. [Crossref] [PubMed]
- Cao P, Jeon J, Levy DT, et al. Potential Impact of Cessation Interventions at the Point of Lung Cancer Screening on Lung Cancer and Overall Mortality in the United States. J Thorac Oncol 2020;15:1160-9. [Crossref] [PubMed]
- Meza R, Cao P, Jeon J, et al. Impact of Joint Lung Cancer Screening and Cessation Interventions Under the New Recommendations of the U.S. Preventive Services Task Force. J Thorac Oncol 2022;17:160-6. [Crossref] [PubMed]
- Shelley D, Wang VH, Taylor K, et al. Accelerating integration of tobacco use treatment in the context of lung cancer screening: Relevance and application of implementation science to achieving policy and practice. Transl Behav Med 2022;12:1076-83. [Crossref] [PubMed]
- Stone E, Marshall H. Tobacco cessation in lung cancer screening-do we have the evidence? Transl Lung Cancer Res 2018;7:S270-4. [Crossref] [PubMed]
- Joseph AM, Rothman AJ, Almirall D, et al. Lung Cancer Screening and Smoking Cessation Clinical Trials. SCALE (Smoking Cessation within the Context of Lung Cancer Screening) Collaboration. Am J Respir Crit Care Med 2018;197:172-82. [Crossref] [PubMed]
- Slatore CG, Baumann C, Pappas M, et al. Smoking behaviors among patients receiving computed tomography for lung cancer screening. Systematic review in support of the U.S. preventive services task force. Ann Am Thorac Soc 2014;11:619-27. [Crossref] [PubMed]
- Brain K, Carter B, Lifford KJ, et al. Impact of low-dose CT screening on smoking cessation among high-risk participants in the UK Lung Cancer Screening Trial. Thorax 2017;72:912-8. [Crossref] [PubMed]
- Tremblay A, Taghizadeh N, MacEachern P, et al. Two-Year Follow-Up of a Randomized Controlled Study of Integrated Smoking Cessation in a Lung Cancer Screening Program. JTO Clin Res Rep 2021;2:100097. [Crossref] [PubMed]
- Tammemägi MC, Berg CD, Riley TL, et al. Impact of lung cancer screening results on smoking cessation. J Natl Cancer Inst 2014;106:dju084. [Crossref] [PubMed]
- Townsend CO, Clark MM, Jett JR, et al. Relation between smoking cessation and receiving results from three annual spiral chest computed tomography scans for lung carcinoma screening. Cancer 2005;103:2154-62. [Crossref] [PubMed]
- Tremblay A, Taghizadeh N, Huang J, et al. A Randomized Controlled Study of Integrated Smoking Cessation in a Lung Cancer Screening Program. J Thorac Oncol 2019;14:1528-37. [Crossref] [PubMed]
- Taylor KL, Williams RM, Li T, et al. A Randomized Trial of Telephone-Based Smoking Cessation Treatment in the Lung Cancer Screening Setting. J Natl Cancer Inst 2022;114:1410-9. [Crossref] [PubMed]
- Leone FT, Zhang Y, Evers-Casey S, et al. Initiating Pharmacologic Treatment in Tobacco-Dependent Adults. An Official American Thoracic Society Clinical Practice Guideline. Am J Respir Crit Care Med 2020;202:e5-e31. [Crossref] [PubMed]
- Lopes LC, Zhang Y, Ross S, et al. Varenicline for Tobacco-Dependent Adults Who Are Not Ready to Discontinue Use: A Systematic Review and Meta-Analysis. Ann Am Thorac Soc 2022;19:2077-86. [Crossref] [PubMed]
- Thomas NA, Ward R, Tanner NT, et al. Factors Associated With Smoking Cessation Attempts in Lung Cancer Screening: A Secondary Analysis of the National Lung Screening Trial. Chest 2023;163:433-43. [Crossref] [PubMed]
- Heffner JL, Coggeshall S, Wheat CL, et al. Receipt of Tobacco Treatment and One-Year Smoking Cessation Rates Following Lung Cancer Screening in the Veterans Health Administration. J Gen Intern Med 2022;37:1704-12. [Crossref] [PubMed]
- Pastorino U, Ladisa V, Trussardo S, et al. Cytisine Therapy Improved Smoking Cessation in the Randomized Screening and Multiple Intervention on Lung Epidemics Lung Cancer Screening Trial. J Thorac Oncol 2022;17:1276-86. [Crossref] [PubMed]
- Taghizadeh N, Taylor KL, MacEachern P, et al. Tobacco use and motivation to stop smoking among long-term smokers who are ineligible for lung cancer screening. Lung Cancer 2017;111:101-7. [Crossref] [PubMed]
- Seijo LM, Peled N, Ajona D, et al. Biomarkers in Lung Cancer Screening: Achievements, Promises, and Challenges. J Thorac Oncol 2019;14:343-57. [Crossref] [PubMed]
Cite this article as: Baeza SM, Lam S. Lung cancer screening—contemporary issues: a narrative review. Curr Chall Thorac Surg 2023;5:47.