A review of managing catastrophes in robotic-assisted thoracic surgery
Introduction
Lung cancer is the most commonly diagnosed cancer worldwide, with over 2.5 million new cases, accounting for approximately 1 in 8 of all cancers diagnosed globally.
It is also the leading cause of cancer related mortality in the United States each year (1). For early-stage non-small cell lung cancer (NSCLC), lung resection with anatomic lobectomy is often the standard of care (2). Robot-assisted lobectomy is becoming a popular alternative to video-assisted thoracoscopic surgery (VATS) lobectomy and open thoracotomy (3). Compared to open thoracotomy, VATS and robot-assisted lobectomies are associated with a lower incidence of arrhythmia, shorter hospital length of stay, less postoperative pain, and reduced morbidity and mortality (3-5). Intraoperative complications and the surgeon’s learning curve are major barriers to the adoption of robotic techniques (2,6). Furthermore, the surgeon’s comfort with the robot also dictates the ability and success with which any encountered injuries are evaluated and repaired.
Catastrophic complications are often defined as necessitating emergent thoracotomy or an additional unplanned major surgical intervention (5). Although robot-assisted surgery offers good visibility, three-dimensional imaging, and precise movements, the lack of tactile feedback can be a contributing factor, leading to complications (6). The three categories of catastrophic injuries during anatomic lobectomies typically involve one or more of the following structures: bronchus, pulmonary arteries, or pulmonary veins (5,6).
Intraoperative catastrophes during lung resections, though infrequent, pose significant challenges and can dramatically impact patient outcomes. The incidence of such events, including pulmonary artery injuries and other major vascular complications, has been reported to be low but with substantial consequences, such as increased perioperative mortality and longer operative durations (7,8). Effective management strategies are crucial, often involving immediate surgical interventions such as pressure control, fibrin sealant application, or conversion to thoracotomy (7,9). Identifying risk factors—such as higher clinical tumor-node-metastasis (TNM) stage, lower forced expiratory volume, and preoperative radiotherapy—can aid in preoperative planning and risk stratification (7). Technological advances, particularly in robotic and video-assisted thoracoscopic surgeries, have introduced new challenges and management strategies, emphasizing the need for continuous awareness and structured action plans to mitigate risks (7,9).
Current literature focuses on confirming the efficacy of robotic surgery compared to previous modalities such as thoracoscopy or thoracotomy, but there is a current gap in a review of potential complications and their management in robotic-assisted lobectomy. This review aims to synthesize current knowledge on the incidence, management strategies, risk factors, surgeon experience, and technological advances related to intraoperative catastrophes in lung resections, providing a comprehensive resource for thoracic surgeons.
This article discusses the various complications associated with robot-assisted lobectomies, including tracheobronchial, pulmonary artery, and pulmonary vein injuries. It explores the management strategies for these catastrophic complications and emphasizes the importance of the surgeon’s experience and comfort with robotic systems. The article also addresses the impact of neoadjuvant immunotherapy on lung cancer surgery, highlighting the challenges and potential benefits of integrating these treatments. Additionally, it examines the challenges presented by fused fissures, which occur in up to 24% of patients, particularly between the right upper lobe and right middle lobe.
Tracheobronchial Injuries
Tracheobronchial injuries are potentially life-threatening and often result from blunt, penetrating, or iatrogenic injury (10). The incidence of airway injury during endotracheal intubation is about 0.005%, increasing to 0.05–0.19% with double-lumen intubations. These injuries typically occur in the midline and the lower third of the trachea (11). Approximately 0.2% of robotic anatomical lung resections result in airway injury (7). Risk factors for tracheal injuries during intubation include chronic corticosteroid use, obesity, short obese postmenopausal females, emergency intubation, manipulation of the tube with an inflated cuff, and late removal of guide rods during intubation (12,13). Intraoperative tracheobronchial injuries can occur during any intrathoracic surgery and have been described in association with lung resection, esophagectomy, empyema surgery, and mediastinal lymph node dissection.
Intraoperatively, airway injuries during dissection are significant and not uncommon. According to Takase et al., bronchial injuries occurred in 2.2% of patients undergoing robotic assisted anatomical pulmonary resection for lung cancer. These injuries were typically managed by primary repair (9).
Risk factors
Risk factors for intraoperative tracheobronchial injuries during robotic surgeries can include both anatomical complexities and patient specific factor. Upper lobectomies, which involves more complex anatomical region, are associated with longer operative times and higher complication rates (9,14). Also the presence of fused fissures or incomplete interlobar fissures increases the risk of transection of bronchovascular structures, including the tracheobronchial tree, during dissection of lymphnodes (9,14). Furthermore, patients factors such as current smoking and severe airflow obstruction are significant risk factors. American Association for Thoracic Surgery notes that current smoking, particularly in patients with severe airflow obstruction (GOLD stage 2/3), is associated with higher rates of postoperative complications such as prolonged air leak and pneumonia, which can be indicative of underlying airway injuries (15). Furthermore, patients with reduced pulmonary function, such as those with a forced expiratory volume in 1 second (FEV1) ≤60% or diffusing capacity for carbon monoxide (DLCO) ≤50%, are at increased risk for pulmonary complications, including tracheobronchial injuries (16).
Clinical presentation and diagnosis
Early recognition of tracheal injury is important for good outcomes. The method and extent of injury determine the patient’s signs and symptoms. Small injuries that are not recognized may heal on their own, although some lead to tracheal stenosis or fistula.
Tracheobronchial injuries frequently present with symptoms such as subcutaneous emphysema, dyspnea and hemoptysis. Once the patient is extubated, symptoms such as persistent cough, dyspnea, desaturations, and severe pain are highly suspicious for tracheal injury (17).
Tracheal injuries are usually recognized immediately in the intraoperative setting, as the injury is often visible within the operative field. However, injuries due to thermal injuries or during dissection of lymph nodes may be unrecognized.
The symptoms may be subtle and are masked intraoperatively by the effect of anesthesia. Intraoperative signs such as ventilatory resistance, air leaks from the cuff, and bloody secretions can rise the suspicion of a traumatic tracheobronchial injury.
Hulscher et al. (18) reported on six patients who experienced tracheal injuries during esophagectomy surgeries. In five instances, the injury was identified during the operation, while in the sixth patient, it was detected on the first day after surgery. This patient had self-extubated, and upon reintubation, air leakage was observed from the cervical incision site. Similarly, Bartels and colleagues (19) described 31 cases of tracheobronchial injuries discovered postoperatively following esophagectomy. Among these patients, eight presented with pneumomediastinum, 19 with pneumothorax, and four developed a tracheoesophageal fistula.
The diagnosis can be confirmed with direct visualization, bronchoscopy, or saline submersion test.
Management and repair
It has been proposed that injuries to the trachea can be classified morphologically according to the tracheal layers injured, which can guide non-operative management. Levels I & II are those without complete disruption of the posterior tracheal membrane. Level IIIa describes complete laceration of the membrane. Level IIIb includes additional injury to the esophagus or severe mediastinitis (20). However, the main factors that guide operative vs. non-operative management are the size of the injury, the degree of pneumothorax or air leakage, and the condition of the patient.
Intraoperative repair of tracheal injuries begins with airway control. Intubated patients with suspected tracheal injuries should have urgent flexible or rigid bronchoscopy while the endotracheal tube is being pulled backward to visualize the lesion to its proximal end. The endotracheal tube cuff can be positioned distal to the lesion. However, if a tracheal tear is detected intraoperatively during cardiothoracic surgery, it is best treated surgically even if it was caused by intubation (11).
For small, clean lacerations (Figure 1), direct suturing using robotic instruments is often the first-line approach. This involves precise placement of sutures to close the defect (9,17). Intraoperatively, running or interrupted absorbable sutures (Video 1), such as polyglactin, are typically used for repair (21). There is some evidence for a pedicled muscle flap, especially with wide local ischemia, to reduce the risk of tracheobronchial fistula formation (17). If a stapler does not fire appropriately, the bronchus staple line can be oversewn and buttressed with mediastinal fat as well (9). If one develops a membranous bronchial injury, a bovine pericardial patch can be considered for repair. If a primary repair or patch is not possible, for injuries that are difficult to access or in patients who are not suitable for extensive surgery, endoscopic stenting can be used to bridge the defect and maintain airway patency (11,17).
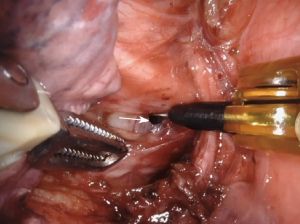
Management strategies differ based on the location of the injury. Central or proximal injuries involving the trachea and main bronchi often require more complex interventions such as primary repair or tracheal resection with end-to-end anastomosis. Distal injuries involving lobar or segmental bronchi may be managed with limited resections or segmentectomy if the airway cannot be repaired (22,23). If unable to repair, the next step is to resect the lobe distal to the injury. If the repair cannot be performed robotically, conversion to open surgery may be necessary.
Conservative management is reserved for stable patients who are not exhibiting symptoms related to the injury. For level I & II injuries and patients with short lesions that can be bypassed with an endotracheal tube, antitussive medications or bypassing the lesion with an endotracheal tube for ventilated patients would be the preferred approach (17). Temporary stent placement to bridge the injury and allow for healing has been described in a few case reports with good results (24-26). Tracheostomy has also been reported as an alternative to open surgical repair by bypassing the lesion and decreasing intraluminal pressure, allowing for healing (27). Tracheostomy is particularly useful in patients who are not ideal candidates for extensive surgical repair due to comorbidities or hemodynamic instability.Furthermore, if the injury is recognized before the start of surgery, Stent placement, especially with silicone or hybrid stents, can provide effective stabilization and promote healing of the airway without the need for more invasive surgical interventions (11). For full-thickness IIIa injuries, stent therapy is well-accepted and often successful in promoting healing while maintaining airway patency (24-26). In such cases, proceeding with the planned lung resection may not be advisable. Aborting the lung resection surgery allows for the tracheobronchial injury to be managed nonoperatively or with minimally invasive techniques, reducing the risk of further complications.
Finally, strategies for ventilation and tube management in tracheobronchial injury include endobronchial intubation, lung-protective ventilation, and high-frequency percussive ventilation (HFPV). Endobronchial intubation involves placing the endotracheal tube distal to the injury site to bypass the lesion, while lung-protective ventilation uses low tidal volumes and low airway pressures to minimize further damage (28). HFPV can aid in lung recruitment and airway clearance without disrupting the healing trachea. Extracorporeal membrane oxygenation (ECMO) plays a critical role in severe cases, providing veno-venous ECMO (VV-ECMO) support to ensure adequate oxygenation and carbon dioxide removal, allowing the lungs to rest and facilitating complex airway surgeries or nonoperative management (29).
Pulmonary artery injury
The most common catastrophic intraoperative event during robotic pulmonary resection is injury to the pulmonary artery (7). In high-volume robotic lobectomy centers, the rate of pulmonary artery injury is estimated to be about 1.5% (30). These injuries occur at a rate of about 1.5% for segmentectomy and 2.6% for lobectomy (30). The right and left upper lobes are the most common locations due to multiple arterial branches in the left upper lobe and the large truncus branch in the right upper lobe. However, the increased incidence could be due to the increased incidence of lung cancer in the upper lobes (31,32). Overall, there is a 6.2% conversion-to-open rate for robotic pulmonary procedures, with 1.1% of all cases being conversion secondary to bleeding events (30). A greater percentage of catastrophic events are caused by inexperienced surgeons, defined as those with fewer than 20 cases (7). The most common contributing factors to pulmonary vessel injury are adherent hilar lymphadenopathy, fibrotic lymph nodes after neoadjuvant therapy, granulomatous disease, and calcified bulky lymph nodes (2,7). Injuries usually occur during dissection of hilar structures particularly when the artery is posterior to the structure (33). The use of energy devices near the pulmonary artery requires extreme cautions to avoid inadvertent contact injury. Injuries can also occur when the artery itself being dissected. Excessive force during retraction can cause tearing to the vessel. Bleeding can occur suddenly and may be significant if the dissection or staple passage exacerbate the injury.
The five “Ps” provide a comprehensive strategy for managing vascular injury in robotic pulmonary resection: prevention, preparedness, poise, pressure, and proximal control (30). Prevention involves careful dissection, identification of key anatomical structures, and minimizing the use of energy devices near the pulmonary artery to avoid injuries. Preparedness requires having a dedicated surgical team that is well-trained in responding to emergencies, with preoperative planning and simulation exercises to ensure everyone understands their role in case of bleeding. Poise is the surgeon’s ability to remain calm and composed, leading the team through a structured response to the injury.
Pressure refers to the immediate application of steady compression to the bleeding site using a rolled sponge or hemostatic agent, avoiding excessive manipulation that could exacerbate the injury. If there is a concern for pulmonary artery injury, the first step should be immediate compression with a pre-rolled sponge using the most anterior robotic instrument (7). Once appropriate, Pressure control is preferably transferred to the most anterior robotic instrument, while maintaining visualization. If the decision is made to convert to open, the team should coordinate to undock the robot carefully while an assistant maintains pressure using instruments through an accessory port. The camera should remain in position until the chest is opened, providing continuous visualization of the injury. Once the robot is undocked, continue visualization through assistant port while preparing for conversion to open (34). Pressure should be applied for at least one minute (9). Next, any robotic arm that is placing retraction on the artery should be relaxed, and insufflation may also be increased to help tamponade the vessel (9). Other means of compression can be achieved using lung parenchyma, along with bipolar forceps and cadieres (7). Finally, while maintaining compression, allow anesthesia to obtain blood products and resuscitate as needed (7).
Proximal Control emphasizes gaining control of the pulmonary artery or vein proximal to the injury using vessel loops or vascular clamps to stop blood flow, enabling definitive repair of the injury with suturing or stapling. Achieving proximal control of the pulmonary artery and outflow control (e.g., pulmonary vein) can be challenging but is critical in cases of vascular injury. However, in instances of catastrophic bleeding, securing proximal control may not be practical. Attempting to isolate the main pulmonary artery while maintaining effective pressure on the bleeding site is challenging—and nearly impossible when the injury is located at the proximal pulmonary artery near the upper lobe branches. Therefore, it’s important to consider establishing proximal control before any potential injury occurs; for example, if a difficult dissection is anticipated or the case has a high risk of pulmonary artery injury, preemptively securing proximal control can be beneficial. A key strategy is to apply a vessel loop around the proximal pulmonary artery to occlude blood flow and prevent further bleeding (8). This technique involves passing a vessel loop around the proximal portion of the pulmonary artery or vein, double looping it, and applying gentle traction to stop blood flow. If the injury is distal to the main artery or involves a branch, a vascular stapler can be used to ligate the injured branch, allowing for safe completion of the procedure (30). These techniques can be difficult to master and performing them robotically requires careful training and simulation exercises.
The next decision to be made is whether to proceed with an elective thoracotomy immediately. This decision is influenced by several factors, including the patient’s risk factors, the surgeon’s experience, the timing of the injury during the operation, the type and anatomical location of the injury, the remaining complexity of the procedure, and the patient’s hemodynamic status. Cerfolio et al., in a series of 1,304 patients undergoing robotic VATS, including 16 patients with major vascular injuries, demonstrated that if pressure is applied immediately, blood pressure should remain stable with minimal blood loss (31). More experienced surgeons may attempt to repair using minimally invasive techniques. The most conservative and safest option is to perform a thoracotomy to safely address and repair the injury. The primary objective of the surgery shifts from achieving an R0 resection with complete lymph node removal via minimally invasive methods to prioritizing the patient’s survival and performing an R0 resection with full lymph node dissection. If hemostasis is unable to be achieved, the vessel may be clipped, stapled, or repaired with prolene suture which can allow continued VATS approach (7).
If the decision is made to convert to open, all robotic instruments must be completely removed, and bleeding to be controlled solely with sponges, without any external instruments applying pressure. If bleeding persists (which is rare), pressure should be applied using a non-robotic instrument through the access port by a bedside assistant while the chest is opened safely and calmly (31). Inserting a video assisted camera while ensures that the vessel is not bleeding while actively opening the chest. The five “Ps” have been utilized to describe the strategy when it comes to vascular injury: Prevention, Preparedness, Poise, Pressure, and Proximal Control (30,31). Given the steep learning curve of robotic surgery, staying robotic for repair may prove more difficult, and the injury can be worsened if the surgeon is not experienced with robotic techniques (35). If hemostasis is unsuccessful, or there is concern for hemodynamic instability, pressure must be maintained from an assist port while the robot is undocked and conversion to thoracotomy is completed (7,30). Having in place a plan, and even performing practice scenarios with the team, for when bleeding occurs can improve outcomes.
Pulmonary vein injury
The next most common catastrophic intraoperative event during robotic pulmonary resection is injury to the pulmonary vein (30). Once again, the five “Ps” strategy—Prevention, Preparedness, Poise, Pressure, and Proximal Control—is paramount to follow (30). Given their lower pressure state, pulmonary vein injuries are typically controlled with pressure. Aggressive suction should be avoided due to concern for air embolism development (30). Aggressive and repetitive suctioning leads to lowering of intrathoracic pressure followed by compensatory high flow insufflation. This leads to transient intrathoracic pressure exceeding systemic venous pressure which in turn increases the risk of air embolism through injured vein (36). Pressure should be held for one minute while a tightly rolled sponge with a fibrin sealant is introduced, which can be held in place for up to three minutes (30). Similar to pulmonary artery injury, while maintaining compression, allow anesthesia to obtain blood products and resuscitate as needed (7). If hemostasis is unable to be achieved, the vessel may be repaired with prolene suture or divided with a stapler (7,30). If hemostasis is unsuccessful, or there is concern for hemodynamic instability, pressure must be maintained from an assist port while the robot is undocked and conversion to thoracotomy is completed (7,30).
It is important to consider the surgeon’s experience in robotic repair; conversion to open surgery would be the safest option before attempting robotic repair for those who are not as experienced with the robot. Furthermore, hybrid robotic and mini-thoracotomy approaches have been described, which may be another strategy if struggling robotically, or if performing a large thoracotomy, which may be more morbid for the patient (37).
Lung resection following immunotherapy
NSCLC accounts for about 85% of lung cancer cases (38). Although surgery and adjuvant therapies such as chemotherapy and radiotherapy are standard treatments for early-stage NSCLC (39-41), achieving complete removal of advanced-stage tumors (stage III, N2/N3) remains difficult due to tumor size, location, and involvement of metastatic lymph nodes. These challenges result in high recurrence and mortality rates even after surgery. Recently, neoadjuvant immunotherapy has emerged as a promising approach, offering new options for treating resectable NSCLC by enhancing the likelihood of radical tumor removal and improving patient outcomes (42).
In recent years, numerous clinical trials have investigated neoadjuvant immunotherapy for NSCLC, with some yielding promising results (43-48). For instance, the phase 3 CheckMate-816 trial demonstrated that combining neoadjuvant nivolumab with chemotherapy significantly extends event-free survival and increases the percentage of patients achieving a pathological complete response (pCR) compared to chemotherapy alone (45).
There are still no clear established guidelines for the timing of surgery after immunotherapy. Pre-operative work-up is important to understand the involvement of major structures such as the pulmonary artery and vein (49). Some studies have decided on about 3–6 weeks as the timeline to wait after the last treatment session (11,50). The rationale is that of balance between poor wound healing if surgery is undertaken too soon versus dense fibrosis setting in if surgery is delayed too long (51).
Nevertheless, challenging surgical procedures following neoadjuvant immunotherapy have frequently been reported in clinical practice. For example, the KEYNOTE-671 trial documented that up to 32 out of 397 patients could not undergo complete resection after immunotherapy (52). Antecedent studies have also suggested that neoadjuvant immunotherapy significantly impacts thoracic surgery (53-55). These studies described perioperative conditions, particularly intraoperative challenges and postoperative complications, but did not analyze them in detail due to small cohort sizes (56). Some studies have shown that neoadjuvant chemoimmunotherapy and smoking history are independent risk factors for challenging surgeries and complications of Clavien-Dindo class II or higher. Conversion to open surgery is often a consequence of these challenging conditions (11,57).
It is widely believed that immune checkpoint inhibitors (ICIs) and the resulting chronic inflammatory reaction can cause tissue adhesion and fibrosis, increasing the difficulty of resection. However, the factors contributing to this process remain to be further elucidated. The TOP 1501 trial investigated the safety and efficacy of neoadjuvant pembrolizumab in patients with untreated clinical stage IB to IIIA NSCLC, administering two cycles of pembrolizumab before surgery and four cycles post-surgery, with optional adjuvant chemotherapy and radiation (48). A lower proportion of patients in TOP 1501 underwent minimally invasive surgery. This may be due to intense fibrosis at the tumor site and involved nodal stations, as noted by Bott and colleagues in their study of surgery after neoadjuvant nivolumab (53).
Most adhesions are around the lymph nodes, making surgery particularly difficult for patients with N1+. For instance, a positron emission tomography (PET) scan revealing an N1 node on the A1 branch during a left upper lobe lobectomy exemplifies these challenges. The inflammation of lymph nodes in these patients resembles that seen in infections like coccidioidomycosis or histoplasmosis, but without the typical calcifications. During surgery, meticulous dissection of the lymph nodes is necessary, and surgeons should look for clear anatomical planes on the pulmonary artery to avoid complications. In some cases, reconstructive surgery with pulmonary arterioplasty may be required due to fibrosis and adhesions. If minimally invasive techniques prove challenging, converting to an open surgical approach is not considered a failure but rather a necessary step to ensure patient safety and successful tumor removal.
Strengths and limitations
This review provides a comprehensive synthesis of current knowledge on the management of catastrophic complications during robotic-assisted thoracic surgery, addressing a significant gap in existing literature. By consolidating evidence from various studies, we offer practical guidance for surgeons to improve intraoperative management strategies.
However, the review is limited by the scarcity of large-scale, high-quality studies specifically focusing on catastrophic events in robotic thoracic surgery. Much of the available data comes from single-center experiences or small cohorts, which may limit the generalizability of our findings. Additionally, rapid advancements in surgical technology and techniques may outpace some of the strategies discussed. Future research should focus on multicenter studies and the development of standardized protocols to further enhance surgical safety and patient outcomes.
Conclusions
In conclusion, while robot-assisted lobectomy has emerged as a promising alternative to traditional surgical methods due to its reduced postoperative complications and enhanced visualization, ergonomics, and instrumentation, intraoperative complications remain a significant barrier to its wider adoption. The steep learning curve of robotic surgery can be addressed through hybrid approaches that help surgeons develop the necessary skills. Catastrophic events such as tracheobronchial injuries and pulmonary artery or vein injuries necessitate meticulous preoperative and intraoperative management, with strategies like the five “Ps”—Prevention, Preparedness, Poise, Pressure, and Proximal Control—being essential for effective management. Neoadjuvant immunotherapy introduces new complexities due to tissue adhesions and fibrosis, complicating resections; however, clinical trials like CheckMate-816 and KEYNOTE-671 demonstrate potential benefits when combining immunotherapy with traditional treatments.
This paper provides surgeons with practical tips and techniques to manage catastrophic complications and prevent conversion to thoracotomy, underscoring that calling for help and converting to open surgery when necessary are crucial steps to ensure patient safety and successful outcomes.
Acknowledgments
Funding: None.
Footnote
Peer Review File: Available at https://ccts.amegroups.com/article/view/10.21037/ccts-24-30/prf
Conflicts of Interest: All authors have completed the ICMJE uniform disclosure form (available at https://ccts.amegroups.com/article/view/10.21037/ccts-24-30/coif). S.G.W. is a speaker for Intuitive Surgical. O.T.O. is a speaker for Intuitive Surgical, Medtronic, Atricure, and Johnson & Johnson (J&J). The other authors have no conflicts of interest to declare.
Ethical Statement: The authors are accountable for all aspects of the work in ensuring that questions related to the accuracy or integrity of any part of the work are appropriately investigated and resolved. All clinical procedures described in this study were performed in accordance with the ethical standards of the institutional and/or national research committee(s) and with the Helsinki Declaration (as revised in 2013). Written informed consent was obtained from the patients for the publication of this article, accompanying image and video.
Open Access Statement: This is an Open Access article distributed in accordance with the Creative Commons Attribution-NonCommercial-NoDerivs 4.0 International License (CC BY-NC-ND 4.0), which permits the non-commercial replication and distribution of the article with the strict proviso that no changes or edits are made and the original work is properly cited (including links to both the formal publication through the relevant DOI and the license). See: https://creativecommons.org/licenses/by-nc-nd/4.0/.
References
- Bray F, Laversanne M, Sung H, et al. Global cancer statistics 2022: GLOBOCAN estimates of incidence and mortality worldwide for 36 cancers in 185 countries. CA Cancer J Clin 2024;74:229-63. [Crossref] [PubMed]
- Herrera LJ, Schumacher LY, Hartwig MG, et al. Pulmonary Open, Robotic, and Thoracoscopic Lobectomy study: Outcomes and risk factors of conversion during minimally invasive lobectomy. J Thorac Cardiovasc Surg 2023;166:251-262.e3. [Crossref] [PubMed]
- Oh DS, Reddy RM, Gorrepati ML, et al. Robotic-Assisted, Video-Assisted Thoracoscopic and Open Lobectomy: Propensity-Matched Analysis of Recent Premier Data. Ann Thorac Surg 2017;104:1733-40. [Crossref] [PubMed]
- Paul S, Altorki NK, Sheng S, et al. Thoracoscopic lobectomy is associated with lower morbidity than open lobectomy: a propensity-matched analysis from the STS database. J Thorac Cardiovasc Surg 2010;139:366-78. [Crossref] [PubMed]
- Onaitis MW, Petersen RP, Balderson SS, et al. Thoracoscopic lobectomy is a safe and versatile procedure: experience with 500 consecutive patients. Ann Surg 2006;244:420-5. [Crossref] [PubMed]
- van der Sluis PC, Schizas D, Liakakos T, et al. Minimally Invasive Esophagectomy. Dig Surg 2020;37:93-100. [Crossref] [PubMed]
- Cao C, Cerfolio RJ, Louie BE, et al. Incidence, Management, and Outcomes of Intraoperative Catastrophes During Robotic Pulmonary Resection. Ann Thorac Surg 2019;108:1498-504. [Crossref] [PubMed]
- Decaluwe H, Petersen RH, Hansen H, et al. Major intraoperative complications during video-assisted thoracoscopic anatomical lung resections: an intention-to-treat analysis. Eur J Cardiothorac Surg 2015;48:588-98; discussion 599. [Crossref] [PubMed]
- Takase Y, Miyajima M, Chiba Y, et al. Causes and management of intraoperative complications in robot-assisted anatomical pulmonary resection for lung cancer. J Thorac Dis 2022;14:3221-33. [Crossref] [PubMed]
- Kiser AC, O'Brien SM, Detterbeck FC. Blunt tracheobronchial injuries: treatment and outcomes. Ann Thorac Surg 2001;71:2059-65. [Crossref] [PubMed]
- Grewal HS, Dangayach NS, Ahmad U, et al. Treatment of Tracheobronchial Injuries: A Contemporary Review. Chest 2019;155:595-604. [Crossref] [PubMed]
- Miñambres E, Burón J, Ballesteros MA, et al. Tracheal rupture after endotracheal intubation: a literature systematic review. Eur J Cardiothorac Surg 2009;35:1056-62. [Crossref] [PubMed]
- Conti M, Pougeoise M, Wurtz A, et al. Management of postintubation tracheobronchial ruptures. Chest 2006;130:412-8. [Crossref] [PubMed]
- Tanaka Y, Tane S, Doi T, et al. Factors affecting the short-term outcomes of robotic-assisted thoracoscopic surgery for lung cancer. Surg Today 2024;54:874-81. [Crossref] [PubMed]
- Pennathur A, Brunelli A, Criner GJ, et al. Definition and assessment of high risk in patients considered for lobectomy for stage I non-small cell lung cancer: The American Association for Thoracic Surgery expert panel consensus document. J Thorac Cardiovasc Surg 2021;162:1605-1618.e6. [Crossref] [PubMed]
- Cao C, Louie BE, Melfi F, et al. Impact of pulmonary function on pulmonary complications after robotic-assisted thoracoscopic lobectomy. Eur J Cardiothorac Surg 2020;57:338-42. [Crossref] [PubMed]
- Welter S, Essaleh W. Management of tracheobronchial injuries. J Thorac Dis 2020;12:6143-51. [Crossref] [PubMed]
- Hulscher JB, ter Hofstede E, Kloek J, et al. Injury to the major airways during subtotal esophagectomy: incidence, management, and sequelae. J Thorac Cardiovasc Surg 2000;120:1093-6. [Crossref] [PubMed]
- Bartels HE, Stein HJ, Siewert JR. Tracheobronchial lesions following oesophagectomy: prevalence, predisposing factors and outcome. Br J Surg 1998;85:403-6. [Crossref] [PubMed]
- Cardillo G, Carbone L, Carleo F, et al. Tracheal lacerations after endotracheal intubation: a proposed morphological classification to guide non-surgical treatment. Eur J Cardiothorac Surg 2010;37:581-7. [Crossref] [PubMed]
- Zhao Z, Zhang T, Yin X, et al. Update on the diagnosis and treatment of tracheal and bronchial injury. J Thorac Dis 2017;9:E50-6. [Crossref] [PubMed]
- Boutros J, Marquette CH, Ichai C, et al. Multidisciplinary management of tracheobronchial injury. Eur Respir Rev 2022;31:210126. [Crossref] [PubMed]
- Morita M, Saeki H, Okamoto T, et al. Tracheobronchial fistula during the perioperative period of esophagectomy for esophageal cancer. World J Surg 2015;39:1119-26. [Crossref] [PubMed]
- Lee BE, Korst RJ. Successful Treatment of an Iatrogenic Tracheal Laceration With a Temporary Polyurethane-Coated Nitinol Stent. Ann Thorac Surg 2016;102:e11-2. [Crossref] [PubMed]
- Marchese R, Mercadante S, Paglino G, et al. Tracheal stent to repair tracheal laceration after a double-lumen intubation. Ann Thorac Surg 2012;94:1001-3. [Crossref] [PubMed]
- Yamamoto S, Endo S, Endo T, et al. Successful silicon stent for life-threatening tracheal wall laceration. Ann Thorac Cardiovasc Surg 2013;19:49-51. [Crossref] [PubMed]
- Ovári A, Just T, Dommerich S, et al. Conservative management of post-intubation tracheal tears-report of three cases. J Thorac Dis 2014;6:E85-91. [PubMed]
- Abrams D, Agerstrand C, Beitler JR, et al. Risks and Benefits of Ultra-Lung-Protective Invasive Mechanical Ventilation Strategies with a Focus on Extracorporeal Support. Am J Respir Crit Care Med 2022;205:873-82. [Crossref] [PubMed]
- Clark J, Morrison JJ, O'Connor JV. Extracorporeal Membrane Oxygenation Support During Repair of a Noniatrogenic Tracheal Injury. Ann Thorac Surg 2022;113:e49-51. [Crossref] [PubMed]
- Gharagozloo F, Meyer M. Technique of robotic lobectomy III: control of major vascular injury, the 5 “P”’s. Mini-invasive Surg 2020;4:57. [Crossref]
- Cerfolio RJ, Bess KM, Wei B, et al. Incidence, Results, and Our Current Intraoperative Technique to Control Major Vascular Injuries During Minimally Invasive Robotic Thoracic Surgery. Ann Thorac Surg 2016;102:394-9. [Crossref] [PubMed]
- Decaluwe H, Petersen RH, Hansen H, et al. Major intraoperative complications during video-assisted thoracoscopic anatomical lung resections: an intention-to-treat analysis. Eur J Cardiothorac Surg 2015;48:588-98; discussion 599. [Crossref] [PubMed]
- Berry MF. Pulmonary Artery Bleeding During Video-Assisted Thoracoscopic Surgery: Intraoperative Bleeding and Control. Thorac Surg Clin 2015;25:239-47. [Crossref] [PubMed]
- Stanley MD, Sancheti MS. Management of Complications in Robotic Thoracic Surgery. Thorac Surg Clin 2023;33:19-24. [Crossref] [PubMed]
- Pagès PB, Gutierrez I, Baste JM. Pulmonary artery repair during robotic lung resection: narrative review and management. J Vis Surg 2022;8:3. [Crossref]
- Shimizu K, Usuda M, Kakizaki Y, et al. Cerebral infarction by paradoxical gas embolism detected after laparoscopic partial hepatectomy with an insufflation management system: a case report. Surg Case Rep 2023;9:34. [Crossref] [PubMed]
- Uchida S, Matsunaga T, Takamochi K, et al. Hybrid robotic-assisted and minithoracotomy for pulmonary arterioplasty using autologous pulmonary vein graft. JTCVS Tech 2023;20:166-8. [Crossref] [PubMed]
- Sung H, Ferlay J, Siegel RL, et al. Global Cancer Statistics 2020: GLOBOCAN Estimates of Incidence and Mortality Worldwide for 36 Cancers in 185 Countries. CA Cancer J Clin 2021;71:209-49. [Crossref] [PubMed]
- NSCLC Meta-analyses Collaborative Group. Adjuvant chemotherapy, with or without postoperative radiotherapy, in operable non-small-cell lung cancer: two meta-analyses of individual patient data. Lancet 2010;375:1267-77. [Crossref] [PubMed]
- Herbst RS, Morgensztern D, Boshoff C. The biology and management of non-small cell lung cancer. Nature 2018;553:446-54. [Crossref] [PubMed]
- Sosa MS, Bragado P, Aguirre-Ghiso JA. Mechanisms of disseminated cancer cell dormancy: an awakening field. Nat Rev Cancer 2014;14:611-22. [Crossref] [PubMed]
- Liang W, Cai K, Chen C, et al. Expert consensus on neoadjuvant immunotherapy for non-small cell lung cancer. Transl Lung Cancer Res 2020;9:2696-715. [Crossref] [PubMed]
- Chaft JE, Oezkan F, Kris MG, et al. Neoadjuvant atezolizumab for resectable non-small cell lung cancer: an open-label, single-arm phase II trial. Nat Med 2022;28:2155-61. [Crossref] [PubMed]
- Forde PM, Chaft JE, Pardoll DM. Neoadjuvant PD-1 Blockade in Resectable Lung Cancer. N Engl J Med 2018;379:e14. [Crossref] [PubMed]
- Forde PM, Spicer J, Lu S, et al. Neoadjuvant Nivolumab plus Chemotherapy in Resectable Lung Cancer. N Engl J Med 2022;386:1973-85. [Crossref] [PubMed]
- Gao S, Li N, Gao S, et al. Neoadjuvant PD-1 inhibitor (Sintilimab) in NSCLC. J Thorac Oncol 2020;15:816-26. [Crossref] [PubMed]
- Shu CA, Gainor JF, Awad MM, et al. Neoadjuvant atezolizumab and chemotherapy in patients with resectable non-small-cell lung cancer: an open-label, multicentre, single-arm, phase 2 trial. Lancet Oncol 2020;21:786-95. [Crossref] [PubMed]
- Tong BC, Gu L, Wang X, et al. Perioperative outcomes of pulmonary resection after neoadjuvant pembrolizumab in patients with non-small cell lung cancer. J Thorac Cardiovasc Surg 2022;163:427-36. [Crossref] [PubMed]
- Toker A, Kakuturu J. How I do it: Bronchial sleeve resection and pulmonary angioplasty techniques after induction chemo-immunotherapy. JTCVS Tech 2024;24:202-6. [Crossref] [PubMed]
- Sepesi B, Zhou N, William WN Jr, et al. Surgical outcomes after neoadjuvant nivolumab or nivolumab with ipilimumab in patients with non-small cell lung cancer. J Thorac Cardiovasc Surg 2022;164:1327-37. [Crossref] [PubMed]
- Sasankan P, Hyde J, Wang S, et al. Pulmonary resection after immunotherapy. J Vis Surg 2021;7:29. [Crossref]
- Wakelee H, Liberman M, Kato T, et al. Perioperative Pembrolizumab for Early-Stage Non-Small-Cell Lung Cancer. N Engl J Med 2023;389:491-503. [Crossref] [PubMed]
- Bott MJ, Yang SC, Park BJ, et al. Initial results of pulmonary resection after neoadjuvant nivolumab in patients with resectable non-small cell lung cancer. J Thorac Cardiovasc Surg 2019;158:269-76. [Crossref] [PubMed]
- Deng H, Liu J, Cai X, et al. Radical Minimally Invasive Surgery After Immuno-chemotherapy in Initially-unresectable Stage IIIB Non-small cell Lung Cancer. Ann Surg 2022;275:e600-2. [Crossref] [PubMed]
- Yendamuri S, Groman A, Miller A, et al. Risk and benefit of neoadjuvant therapy among patients undergoing resection for non-small-cell lung cancer. Eur J Cardiothorac Surg 2018;53:656-63. [Crossref] [PubMed]
- Romero Román A, Campo-Cañaveral de la Cruz JL, Macía I, et al. Outcomes of surgical resection after neoadjuvant chemoimmunotherapy in locally advanced stage IIIA non-small-cell lung cancer. Eur J Cardiothorac Surg 2021;60:81-8. [Crossref] [PubMed]
- Bai G, Chen X, Peng Y, et al. Surgery challenges and postoperative complications of lung cancer after neoadjuvant immunotherapy. Thorac Cancer 2024;15:1138-48. [Crossref] [PubMed]
Cite this article as: Battan-Wraith S, Wang K, Elkamel A, Durante K, Conrad H, Iannarelli K, Okusanya OT, Sridhar P, Worrell SG. A review of managing catastrophes in robotic-assisted thoracic surgery. Curr Chall Thorac Surg 2024;6:31.